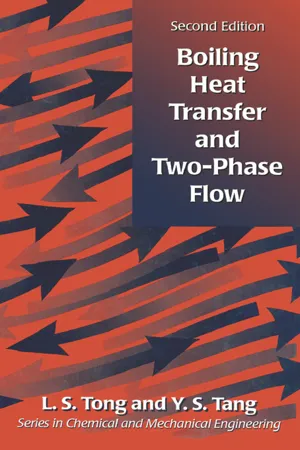
eBook - ePub
Boiling Heat Transfer And Two-Phase Flow
L S Tong
This is a test
Compartir libro
- 572 páginas
- English
- ePUB (apto para móviles)
- Disponible en iOS y Android
eBook - ePub
Boiling Heat Transfer And Two-Phase Flow
L S Tong
Detalles del libro
Vista previa del libro
Índice
Citas
Información del libro
Completely updated, this graduate text describes the current state of boiling heat transfer and two-phase flow, in terms through which students can attain a consistent understanding. Prediction of real or potential boiling heat transfer behaviour, both in steady and transient states, is covered to aid engineering design of reliable and effective systems.
Preguntas frecuentes
¿Cómo cancelo mi suscripción?
¿Cómo descargo los libros?
Por el momento, todos nuestros libros ePub adaptables a dispositivos móviles se pueden descargar a través de la aplicación. La mayor parte de nuestros PDF también se puede descargar y ya estamos trabajando para que el resto también sea descargable. Obtén más información aquí.
¿En qué se diferencian los planes de precios?
Ambos planes te permiten acceder por completo a la biblioteca y a todas las funciones de Perlego. Las únicas diferencias son el precio y el período de suscripción: con el plan anual ahorrarás en torno a un 30 % en comparación con 12 meses de un plan mensual.
¿Qué es Perlego?
Somos un servicio de suscripción de libros de texto en línea que te permite acceder a toda una biblioteca en línea por menos de lo que cuesta un libro al mes. Con más de un millón de libros sobre más de 1000 categorías, ¡tenemos todo lo que necesitas! Obtén más información aquí.
¿Perlego ofrece la función de texto a voz?
Busca el símbolo de lectura en voz alta en tu próximo libro para ver si puedes escucharlo. La herramienta de lectura en voz alta lee el texto en voz alta por ti, resaltando el texto a medida que se lee. Puedes pausarla, acelerarla y ralentizarla. Obtén más información aquí.
¿Es Boiling Heat Transfer And Two-Phase Flow un PDF/ePUB en línea?
Sí, puedes acceder a Boiling Heat Transfer And Two-Phase Flow de L S Tong en formato PDF o ePUB, así como a otros libros populares de Naturwissenschaften y Thermodynamik. Tenemos más de un millón de libros disponibles en nuestro catálogo para que explores.
Información
CHAPTER
ONE
ONE
INTRODUCTION
Boiling heat transfer is defined as a mode of heat transfer that occurs with a change in phase from liquid to vapor. There are two basic types of boiling: pool boiling and flow boiling. Pool boiling is boiling on a heating surface submerged in a pool of initially quiescent liquid. Flow boiling is boiling in a flowing stream of fluid, where the heating surface may be the channel wall confining the flow. A boiling flow is composed of a mixture of liquid and vapor and is the type of two-phase flow that will be discussed in this book. Because of the very high heat transfer rate in boiling, it has been used to cool devices requiring high heat transfer rates, such as rocket motors and nuclear reactors. Its applications in modern industry are so important that large amounts of research in many countries have been devoted to understanding its mechanisms and behavior, especially since the publication of the first edition of this book. The results have not yet been entirely satisfactory in clarifying boiling phenomena and in correlating experimental data on heat transfer in nucleate boiling. This is largely because of the complexity and irreproducibility of the phenomena, caused by the fact that the surface conditions (i.e., the surface roughness, the deposition of foreign materials, or the absorption of gas on the surface) become inherent factors that influence bubble generation (Nishikawa and Fujita, 1990).
1.1 REGIMES OF BOILING
There are several boiling regimes in pool boiling as well as in flow boiling. The only difference lies in the influence of flow effect. The buoyancy effect is significant in a pool boiling system, while the flow forced-convection effect is significant in flow boiling inside a channel.
The various regimes of boiling in a typical case of pool boiling in water at atmospheric pressure are shown in Figure 1.1, which is the conventional log-log representation of heat flux versus wall superheat. These boiling regimes were observed by previous researchers, namely, Leidenfrost (1756), Lang (1888), McAdams et al. (1941), Nukiyama (1934), and Farber and Scorah (1948). In the range A–B (Fig. 1.1), the water is heated by natural convection. With the mechanism of single-phase natural convection, the heat transfer rate q″ is proportional to (ΔTsat)5/4. In the range B–C, the liquid near the wall is superheated and tends to evaporate, forming bubbles wherever there are nucleation sites such as tiny pits or scratches on the surface. The bubbles transport the latent heat of the phase change and also increase the convective heat transfer by agitating the liquid near the heating surface. The mechanism in this range is called nucleate boiling and is characterized by a very high heat transfer rate for only a small temperature difference. There are two subregimes in nucleate boiling: local boiling and bulk boiling. Local boiling is nucleate boiling in a subcooled liquid, where the bubbles formed at the heating surface tend to condense locally. Bulk boiling is nucleate boiling in a saturated liquid; in this case, the bubbles do not collapse. In the nucleate boiling range, q″ varies as (ΔTsat)n, where n generally ranges from 2 to 5. However, the heat flux in nucleate boiling cannot be increased indefinitely. When the population of bubbles becomes too high at some high heat flux point C, the outgoing bubbles may obstruct the path of the incoming liquid. The vapor thus forms an insulating blanket covering the heating surface and thereby raises the surface temperature. This is called the boiling crisis, and the maximum heat flux just before reaching crisis is critical heat flux, which can occur in pool boiling or in various flow patterns of flow boiling (see Sec. 1.3). In the past, the terminology of the boiling crisis was not universal. The pool boiling crisis with constant heat flux supply, or crisis occurring in an annular flow is sometimes called burnout, and that occurring in bubbly flow is sometimes called departure from nucleate boiling (DNB). In this book, all three terms are used interchangeably.
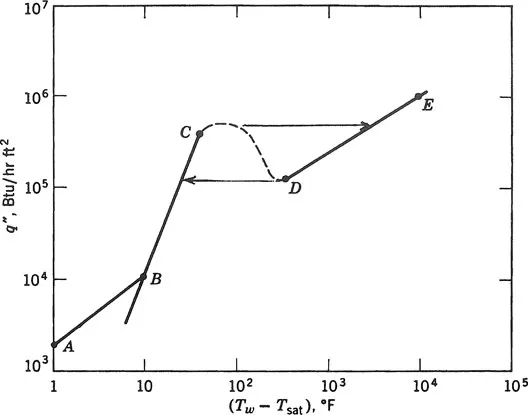
Figure 1.1 Pool boiling regimes: A–B, natural convection; B–C, nucleate boiling; C–D, partial film boiling; D–E, stable film boiling.
In the range C–D, immediately after the critical heat flux has been reached, boiling becomes unstable and the mechanism is then called partial film boiling or transition boiling. The surface is alternately covered with a vapor blanket and a liquid layer, resulting in oscillating surface temperatures. If the power input to the heater is maintained, the surface temperature increases rapidly to point D while the heat flux steadily decreases. In the range D–E, a stable vapor film is formed on the heating surface and the heat transfer rate reaches a minimum. This is called stable film boiling. By further increasing the wall temperature, the heat transfer rate also is increased by thermal radiation. However, too high a temperature would damage the wall. Hence, for practical purposes, the temperature is limited by the material properties.
The boiling regimes mentioned above also exist in flow boiling. The mechanisms are more complicated, however, owing to the fact that two-phase flow plays an important role in the boiling process. For instance, the flow shear may cut off the bubbles from the wall so that the average bubble size is reduced and the frequency is increased. Other interactions between the boiling process and twophase flow are discussed in the next section. As in pool boiling, the range of the boiling curve of interest for most practical applications is that of nucleate boiling (B–C), where very high heat fluxes can be attained at relatively low surface temperatures.
1.2 TWO-PHASE FLOW
Two-phase flows are classified by the void (bubble) distributions. Basic modes of void distribution are bubbles suspended in the liquid stream; liquid droplets suspended in the vapor stream; and liquid and vapor existing intermittently. The typical combinations of these modes as they develop in flow channels are called flow patterns. The various flow patterns exert different effects on the hydrodynamic conditions near the heated wall; thus they produce different frictional pressure drops and different modes of heat transfer and boiling crises. Significant progress has been made in determining flow-pattern transition and modeling.
The microscopic picture of the flow in the proximity of the heated wall can be described in terms of two-phase boundary-layer flow. The macroscopic effect of a two-phase flow on the frictional pressure drop still relies on empirical correlations.
1.3 FLOW BOILING CRISIS
Boiling crisis is a combined phenomenon of hydrodynamics and heat transfer. Owing to excessively high wall temperature, the boiling crisis usually results in damage to the heating surface in a constant-energy-input system. It is imperative, therefore, to predict and prevent the occurrence of the crisis in boiling equipment.
As the flow boiling crisis occurs at a very high heat flux, the prediction of such a crisis has to be closely related with the flow boiling heat transfer, and the appropriate model should also be related with the two-phase flow pattern existing at the CHF conditions. There are two types of parameters by which a flow boiling crisis can be described. One type is the operational parameters of a boiling system, such as system pressure, mass flux, and channel geometry, which are set a priori. An engineering correlation of flow boiling crisis for design purposes can be developed from these parameters, and the parameter effects can be evaluated without revealing the mechanism of the crisis. The other type comprises microscopic parameters such as flow velocity near the wall, local voids, coolant properties, and surface conditions. The latter parameters can be used in calculating the principal forces acting on a bubble or on a control volume. Such data can be useful in modeling the flow pattern, or can be used in organizing significant nondimensional groups to give a phenomenologically meaningful correlation that may reveal the mechanism of the boiling crisis. Early attempts to obtain generalized predictions of flow boiling crises were often based on the assumption that the underlying mechanism was essentially the same as for pool boiling. It has been generally agreed that this is not the case (Tong, 1972; Tong and Hewitt, 1972; Weisman, 1992).
1.4 FLOW INSTABILITY
Flow instability is a phenomenon of combined hydrodynamic and thermodynamic nature and is caused by the large momentum change introduced by boiling of a two-phase flow. It may start with small, constant-amplitude flow oscillations in a channel at low power input. If the power input is increased, the amplitude increases as the flow becomes unstable. Such flow instabilities occurring in boiling equipment can be in the form of instability between parallel channels, flow instability in a natural-circulation loop, or flow instability caused by the difference in pressure drops of interchanging flow patterns. Bouré et al. (1973) classified flow instability phenomena in two categories: static instability and dynamic instability. Within each category there are fundamental (or simple) and compound instabilities. Flow excursion or Ledinegg instability and flow pattern transition instability are examples of fundamental static instabilities, while bumping, geysering, or chugging is a compound relaxation instability. Acoustic oscillations are examples of fundamental dynamic instability, while parallel channel instability is a compound dynamic instability.
Ruddick (1953) and Lowdermilk et al. (1958) found that flow oscillation can induce a premature boiling crisis. Moreover, in a boiling water reactor the flow oscillation may induce a nuclear instability. Thus, in designing a boiling system, it is imperative to predict and prevent those operational conditions that might create flow oscillation.
CHAPTER
TWO
TWO
POOL BOILING
2.1 INTRODUCTION
Pool boiling occurs when a heater is submerged in a pool of initially stagnant liquid. When the surface temperature of the heater exceeds the saturation temperature of the liquid by a sufficient amount, vapor bubbles nucleate on the heater surface. The bubbles grow rapidly in the superheated liquid layer next to the surface until they depart and move out into the bulk liquid. While rising as the result of buoyancy, they either collapse or continue their growth, depending on whether the liquid is locally subcooled or superheated. Thus, in pool boiling, a complex fluid motion around the heater is initiated and maintained by the nucleation, growth, departure, and collapse of bubbles, and by natural convection. A thorough understanding of the process of boiling heat transfer ...