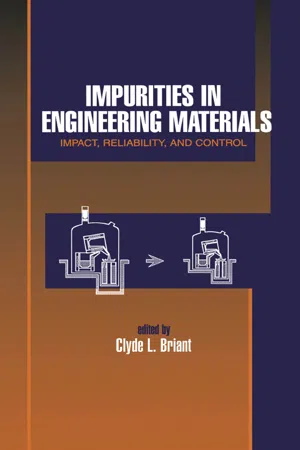
This is a test
- 320 pages
- English
- ePUB (mobile friendly)
- Available on iOS & Android
eBook - ePub
Book details
Book preview
Table of contents
Citations
About This Book
Provides a state-of-the-art account of the various effects of impurities on the properties of engineering alloys. Outlines a wide range of methods for producing cleaner alloys. Traces the technological advances that allow the economical manufacture of purer materials.
Frequently asked questions
At the moment all of our mobile-responsive ePub books are available to download via the app. Most of our PDFs are also available to download and we're working on making the final remaining ones downloadable now. Learn more here.
Both plans give you full access to the library and all of Perlego’s features. The only differences are the price and subscription period: With the annual plan you’ll save around 30% compared to 12 months on the monthly plan.
We are an online textbook subscription service, where you can get access to an entire online library for less than the price of a single book per month. With over 1 million books across 1000+ topics, we’ve got you covered! Learn more here.
Look out for the read-aloud symbol on your next book to see if you can listen to it. The read-aloud tool reads text aloud for you, highlighting the text as it is being read. You can pause it, speed it up and slow it down. Learn more here.
Yes, you can access Impurities in Engineering Materials by Clyde Briant in PDF and/or ePUB format, as well as other popular books in Tecnología e ingeniería & Ciencias de los materiales. We have over one million books available in our catalogue for you to explore.
Information
1
The Need for Clean Materials
Clyde L. Briant
Brown University
Providence, Rhode Island
It has been recognized for many years that the mechanical performance of materials is affected by the presence of impurities. Papers dating from the first half of this century consider this problem and suggest that uncontrolled impurities could be detrimental [1, 2, 3 and 4]. However, during the 1950s and 1960s, as outlined below, concern about the cleanliness of materials became especially great because of the demands of the power generation industry. This interest led to significant research on ways to manufacture clean materials, methods to analyze for impurities, and documentation of the harmful effects of these impurities. This book attempts to summarize this progress and present a picture of where each of these areas of research stands today. The purpose of this introductory chapter is to provide a historical background for this work so that it can be set in the proper perspective.
The term “impurity” can be broadly defined as any unwanted or uncontrolled chemical element present in a material. However, in the examples that we consider below and in the chapters that follow, this definition can be refined. In most alloys that are based on transition metals, impurities will either be those elements from groups IVA-VIA in the periodic table, such as phosphorus, sulfur, tin, and antimony, or elements that are gases in their pure state at room temperature, such as hydrogen, oxygen, or nitrogen. Members of the former group usually come into the material through the raw materials or scrap that are used to make the alloy, and those of the latter group enter the material through the atmosphere. Even in a material that is termed a “dirty” alloy, the bulk concentrations of these elements may seem relatively small; often they are less than 0.1 wt%. However, these elements can combine with the host metal or with other alloying elements in the material to form precipitates that can affect mechanical properties. More importantly, they can segregate to grain boundaries in their elemental form, and at these boundaries their local concentration can become very high. It is these impurities concentrated at the grain boundaries that can lead to intergranular fracture and intergranular corrosion, and it was originally the concern about these two problems that provided the impetus for eliminating impurities from materials.
One of the primary driving forces behind this type of work, as previously stated, came from the power generation industry. In the 1940s the power requirements in the United States and other countries were such that they could be met with the use of small turbines. The integrity of these turbines could be controlled through heat treatment and through extensive inspection programs [5,6]. However, during the 1950s and 1960s the demand for more and more power led manufacturers to design and build larger and larger turbines. These large turbines presented a number of challenges. The first was that their size meant that the mechanical stresses on the turbine were much greater than those that had existed in the past. The second problem was that the turbines were so large that they could not be rapidly quenched and thus have uniform mechanical properties throughout their thickness. The centers of the turbines inevitably cooled more slowly than the surface regions. This slow cooling also allowed time for the impurities to segregate to the grain boundaries and make regions of the material very brittle. Finally, in such a large casting it was impossible to provide uniform composition throughout the piece, and bands of varying chemical composition were observed [5].
The awareness of the embrittlement problem, which is referred to as temper embrittlement (see Chaps. 6 and 7), increased as the steels used to make the newer turbines were developed and tested. The fractures of the embrittled steels were clearly intergranular, but at that time it was not yet possible to obtain chemical analyses of the grain boundaries. Thus, the only recourse was to examine many different heats of materials in which different impurity levels were present [6, 7, 8, 9 and 10]. The results that were obtained were similar to those shown in Figure 1. Here there is a clear correlation between the amount of embrittlement, which is measured as a change in the ductile to brittle transition temperature* and the amount of phosphorus present in the steel. Other results similar to those shown in Figure 1 provided convincing evidence that impurities did cause embrittlement, and therefore it was necessary to develop the technology to produce much cleaner materials.

Figure 1 The change in the ductile to brittle transition temperature plotted as a function of the bulk phosphorus concentration in the steel. (From Ref. 9.)
One way in which the impurity level in these materials could be improved immediately was through the use of higher purity starting materials. This approach was taken, but it was primarily through the development and use of improved melting practices that real improvements were made. The early turbines were prepared with a basic electric furnace, but with the development of vacuum degassing and vacuum deoxidation, followed by electroslag refining and vacuum arc refining, the impurity level in steels decreased significantly. In addition to these general melting procedures, various ladle technologies and the change from single-slag to double-slag melting practices made significant improvement in the cleanliness of the steel [5,11,12]. Figure 2 shows the overall average decrease in phosphorus and sulfur in materials as a function of time as these various technologies were introduced. Figure 3 shows the general increase in fracture toughness that occurred as a result of changes in melting practice.
At the same time that efforts were being made to improve the cleanliness of steels, research was also being performed to improve the overall composition of steel. One of the goals of this work was to develop compositions that would provide adequate hardness throughout a thick section casting such as those required for large turbine applications. Figure 4 shows that the shift from NiMoV steels to NiCrMoV steels caused a significant improvement in the ductile to brittle transition temperature, as did other melting practices. At the same time these compositions proved to be more resistant to temper embrittlement. Figure 5 shows the change in the ductile to brittle transition temperature plotted as a function of exposure time. Ordinarily one would expect that as the exposure temperature increased from approximately 350°C (the range of temperatures used for the NiCr-MoV steels) to approximately 450°C the change in the ductile to brittle transition temperature would increase for a given aging time because segregation would occur more readily. However, the fact that the reverse was seen suggests that the Cr-MoV and the 12Cr-Mo-V-Nb steels are inherently more resistant to temper embrittlement.

Figure 2 The average phosphorus and sulfur concentrations in heavy steel castings for the power generation industry plotted as a function of year. (From Ref. 5.)

Figure 3 Variation in the critical stress intensity factor, KIC, plotted as a function of testing temperature. All steels had a CrMoV base. The conventional steel had higher sulfur than the other two. VCD-vacuum carbon deoxidation melting practice; ESR-electroslag remelt melting practice. (From Ref. 6.)

Figure 4 Trends in the impact toughness of steam turbine rotors. During the 1858–1961 time frame the following changes were made: vacuum pouring, electric furnace melting, the introduction of NiCrMoV steels, water quenching of rotors, and general use of vacuum carbon deoxidation melt practices. (From Ref. 6.)
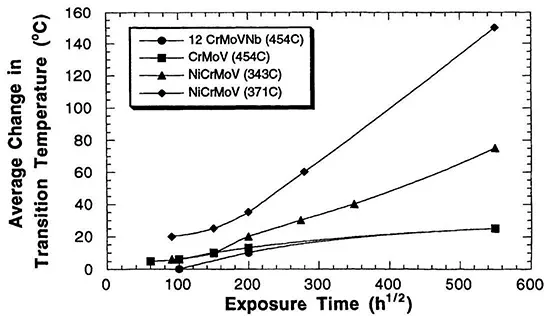
Figure 5 The change in the ductile to brittle transition temperature plotted as a function of exposure time for different steel compositions and temperatures. (From Ref. 5.)
One problem of central concern has been that materials that were put in service would gradually age with time and even at relatively low service temperatures could become embrittled as a result of the diffusion of impurities to the grain boundaries. The data in Figure 5 show that segregation can occur at relatively low temperatures. Figure 6 shows data from material taken from a CrMoV steel rotor that was retired after 17 years of service. One can see that the material that was deembrittled in the laboratory after it was received from the rotor had a significantly higher fracture toughness than the material taken directly from the rotor. One can attribute this change to grain boundary segregation during the life of the rotor.
This same problem of embrittlement during serv...
Table of contents
- Cover Page
- Half title
- Title Page
- Copyright Page
- Contents
- Preface
- Contributors
- 1. The Need for Clean Materials
- 2. Sources of Trace Impurities in Metals
- 3. Preparation of Clean Superalloys
- 4. High Purity, Low Residual, and Clean Steels
- 5. Determination of Trace Impurities in Materials
- 6. Grain Boundary Segregation of Impurities in Iron and Steels and Effects on Steel Properties
- 7. The Effect of Grain Boundary Segregation on Intergranular Failures
- 8. Trace Element Effects on High Temperature Fracture
- 9. Solid Solution Hardening by Impurities
- Index