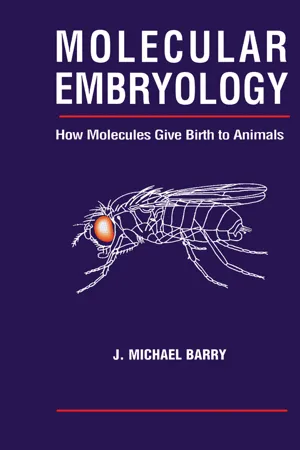
This is a test
- 212 pages
- English
- ePUB (mobile friendly)
- Available on iOS & Android
eBook - ePub
Book details
Book preview
Table of contents
Citations
About This Book
Molecular Embryology explains in simple terms the molecular interactions that transform an egg to a complex embryo that in the end gives rise to a fully-formed animal. In doing so, the book covers one hundred and fifty years of experiments that have led to our present understanding of these molecular interactions. As the text progresses, the reader will gain a sense of the developmental similarities and differences between organisms. Students studying developmental biology and embryology will find this book an extremely useful introduction to the subject and will also appeal to anyone with an interest in the most recent advances in this largely undiscovered territory.
Frequently asked questions
At the moment all of our mobile-responsive ePub books are available to download via the app. Most of our PDFs are also available to download and we're working on making the final remaining ones downloadable now. Learn more here.
Both plans give you full access to the library and all of Perlegoâs features. The only differences are the price and subscription period: With the annual plan youâll save around 30% compared to 12 months on the monthly plan.
We are an online textbook subscription service, where you can get access to an entire online library for less than the price of a single book per month. With over 1 million books across 1000+ topics, weâve got you covered! Learn more here.
Look out for the read-aloud symbol on your next book to see if you can listen to it. The read-aloud tool reads text aloud for you, highlighting the text as it is being read. You can pause it, speed it up and slow it down. Learn more here.
Yes, you can access Molecular Embryology by Michael J. Barry in PDF and/or ePUB format, as well as other popular books in Medicine & Gynecology, Obstetrics & Midwifery. We have over one million books available in our catalogue for you to explore.
Information
CHAPTER ONE
GROWTH OF AN EMBRYO IS FOUNDFOUNDED ON REPEATED CELL DIVISION
DOES A VITAL FORCE DIRECT ANIMAL DEVELOPMENT?
The development of an animal begins when a sperm, which is essentially a mobile package of male genes, fertilizes an egg. The egg contains not only the female genes, but also a complex assembly of molecules needed to initiate development. The transformation of the single cell of the fertilized egg into an adult animal, usually with millions of cells of many different kinds all located in the correct position, is a complex process. Can all these precise changes result from the interaction of the component molecules of the egg according to the normal laws of chemistry? Until recently, many biologists had thought that the answer is no. They believed that an additional vital force must operate in living cells. It is worthwhile first to consider the theories of these âvitalists,â as they are fundamentally related to the theme of this book.
Theories of vitalists that could conceivably be tested by experiment are of two distinct kinds. The first can be illustrated by the ideas of Justus von Liebig,1 a famous German biochemist of the 19th century. In his textbook, Animal Chemistry, he appears to agree with the âmechanistsâ that a living organism results from its component molecules being correctly positioned and that a living organism could, at least in theory, be made in the laboratory. But he believed that when the component molecules are in these positions, a new force of nature manifests itself. This force, he believed, is inherent in all molecules but acts only in the complex structure of the cell. Thus, research in biochemistry would reveal the laws of its action just as experiments in physics revealed the laws of magnetic attraction.
The possibility of a vital force was still entertained in 1945 by the famous physicist, Erwin Schridinger in What Is Life?,2,3 This book made a great impact on physicists and persuaded some to enter biology in the hope ofdiscovering new natural laws. Schrödinger2 concluded, âliving matter, while not eluding the âlaws of physicsâ as established up to date, is likely to involve âother laws of physicsâ hitherto unknown, which, however, once they have been revealed, will form just as integral a part of this science as the formerâ (p. 73). This conclusion was based on his opinion that genes display an inexplicable stability. Schrödinger2 had correctly deduced that a gene is whole or part of a single large molecule, but incorrectly (as we now realize) concluded that these single molecules have a âdurability or permanence that borders on the miraculous.â He mentioned the gene responsible for the famous lip of the Hapsburg dynasty. âHow are we to understand that it has remained unperturbed by the disordering tendency of the heat motion for centuries?â Schrödinger2 compares the biologist to an engineer who is familiar with steam engines but encounters an electric motor for the first time. Although it is made of familiar materials, he suspects that laws that he does not understand are involved in its function. The biologist nevertheless believes that these are comprehensible ordered laws. âHe will not suspect that an electric motor is driven by a ghost because it is set spinning by the turn of a switch, without boiler or steamâ (p. 81).
In contrast, vitalists of the second theory did believe in a ghostly force and that the basis of life lies outside the normal realm of science. Their theories were varied, but the most clearly defined were those of Hans Driesch,4 who died in 1942. He started his career as an embryologist and made fundamental contributions to the subject. But he became convinced that animal development would never be explained solely by the interaction of forces of nature of the kind typically investigated by scientists. As a result, he retired from experimental biology in the early 1900s and became a professor of philosophy at the University of Heidelberg, where he developed his theories of vitalism.
Driesch4 believed that living organisms differ sharply from nonliving organisms in the possession of a vital force unlike any force familiar to scientistsâa purposeful directive force like that suggested by Aristotle. Its purpose is that a living organism should grow to maturity and reproduce. It acts by directing the component parts of the organism along paths they would not take under the sole influence of the normal forces of nature, so causing the organism to develop and function correctly. Driesch claimed that the existence of this force was proved beyond all reasonable doubt by certain experiments in embryology.
For example, when the fertilized egg of a sea urchin develops into an adult, it divides first into two cells; these cells each divide into two more to produce four, and each of these four cells by further division give rise to different parts of the adult. But if the four cells are broken apart and allowed to develop separately, they each form, not different fragments of the adult, but a complete adult sea urchin. Driesch concluded that the four cells are identical and that the normal forces of nature could not cause different parts of an adult to arise from each of four identical cells. (His view has been expressed succinctly by the famous biologist Ernst Mayr,5 âWhat machine, if cut in half, could function normally?â) Again, if the limbs of certain Amphibia are removed, perfect new limbs develop in their place. Driesch considered that this could come about only under the influence of a directive vital force that is attempting to resist damage to the organism and that this could never occur if the component molecules were merely under the sway of the blind forces of nature of the inanimate world. The action of Drieschâs vital force requires that the normal laws of nature can be violated in living organisms, and Driesch considered in detail how this violation be detected.
Even the most famous experimental embryologist of all time, Hans Spemann, a contemporary of Driesch, was undecided about vitalism. As we shall see, Spemannâs experiments form the foundation of our understanding of embryology, and for this work he was awarded the Nobel Prize in 1935. In an address in 1923, he spoke cautiously in relation to Drieschâs work, âI would leave it undecided whether, and how soon, we will encounter ultimately unsolvable problems in the direction taken by Driesch.6â Nevertheless, Spemannâs ideas, like those of most of his contemporaries who were influenced by the impact of the German romantic movement on science, had overtones of vitalism. Thus, in 1938, at the end of a book in English summarizing his lifeâs work, Spemann7 wrote rather obscurely, âthese processes of development, like all vital processes, are comparable, in the way they are connected, to nothing we know in such a degree as to those vital processes of which we have the most intimate knowledge, viz., the psychical ones.... Here and there this intuition is dawning at present. On the way to the high new goal I hope I have made a few steps with these experiments.â
Both vitalist theories can be tested by trying to discover, as biologists do, whether living organisms can be completely explained by the interaction of their component parts according to the familiar laws of chemistry and physics. Over the last 50 years, molecular biologists have shown that most fundamental processes of living cells are founded on the interaction of large molecules according to the normal laws of chemistry. No new natural force of the kind suggested by Liebig or Schrödinger appears to act. But until very recently, there was little experimental evidence that a directive vital force of the kind suggested by Driesch is not required during the development of a fertilized egg into an adult. This evidence is now being provided by the experiments described in this book. As a result, the brain is becoming the last region of the body where it is not entirely unreasonable to suggest that a vital force operates.
THE DIVISION OF EMBRYONIC CELLS
Animals are composed of cells that increase in number by the division of one cell into two (mitosis). Animal development is the transition from the single cell of the fertilized egg to a multicellular adult; hence, cell division is fundamentally involved in the developmental process. This chapter describes some features of cell division that are important in understanding animal development and illustrates these features with details of cell division in the embryos that are most commonly used for research.
The microscopic structure of an animal cell when not dividing is illustrated in Figure 1-1. It is enclosed by the cell membrane. In the center of the cell is the nucleus, which contains the thread-like chromosomes that are largely invisible until cell division. These chromosomes occur in homologous pairs, that is, of identical shape, with the members of each pair being derived from the two parents at fertilization of the egg. The nucleus is enclosed by two closely aligned membranes, and the outer one branches through the cytoplasm (viscous fluid portion of the cell) to form a system of blind tubes, named the endoplasmic reticulum. Outside the endoplasmic reticulum lie the ribosomes, particles that are involved in the formation of proteins. The double nuclear membrane is pierced at many points by nuclear pores, each surrounded by a pore complex of precise structure. The pores enable all but the largest molecules to pass from within the nucleus to the cytoplasm. The cell contains several distinct structures, including nucleoli (usually two) within the nucleus, a centrosome, and many mitochondria and lysosomes in the cytoplasmâall with separate functions. Also, numerous protein enzymes direct the conversion of one kind of molecule into another. Some enzymes are common to all cells, whereas others are peculiar to a particular type of cell.

FIGURE 1-1 Structure of typical nondividing animal cell as revealed by the electron microscope.
Not shown in the diagram is the cytoskeleton, whose filaments ramify throughout the cell and are attached to certain structures. They are important in directing cell division and in causing changes in the movement and shape of cells, which occur repeatedly during animal development. The three kinds of filaments are actin filaments and microtubules, which are continuously degraded and reformed, and the more stable intermediate filaments. Actin filaments are built up within the cell by linking molecules of the protein actin into long chains, which are one molecule thick but coiled into a helix (corkscrew). When associated with filaments composed of the protein myosin, long arrays of actin filaments can contract in length, as they do in muscle cells. A dense network of actin filaments, associated with other proteins, lies beneath the cell membrane to form the cortex, which gives strength to the cell and directs changes in its shape and movement. Actin filaments also branch throughout the cytoplasm. Microtubules are formed by linking molecules of the protein tubulin into long protofilaments; 13 of these protofilaments are aligned side by side into a hollow tube to form the microtubule. The microtubules are less plentiful than actin filaments and are formed in the centrosome (see Fig. 1-1) beside the nucleus and radiate from it. Intermediate filaments, which are composed of fibrous proteins, largely extend from around the nucleus to the cell membrane and appear to stabilize the cell.
The essential requirement of mos...
Table of contents
- Cover
- Half Title
- Title Page
- Copyright Page
- Table of Contents
- Introduction
- Chapter One Growth of An Embryo Is Found on Repeated Cell Division
- Chapter Two How An Embryo Acquires the Correct Shape as Its Cells Divide
- Chapter Three How Differences in Molecular Composition between Embryonic Cells Originate
- Chapter Four Signal Molecules Induce Changes in Cell Composition Throughout Vertebrate Development
- Chapter Five Differences in Embryonic Cell Composition Result from Activation of Different Genes
- Chapter Six Experiments on Drosophila Reveal that Specific Transcription Factors Are Keys that Unlock Embryonic Genes
- Chapter Seven The Frontiers of Research in Molecular Embryology
- Glossary
- Index