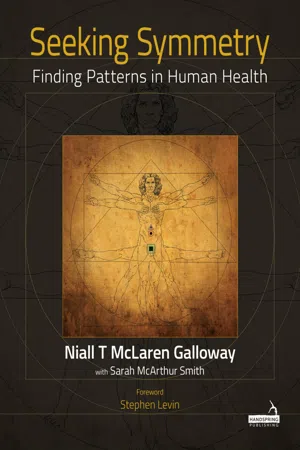
This is a test
- 200 pages
- English
- ePUB (mobile friendly)
- Available on iOS & Android
eBook - ePub
Book details
Book preview
Table of contents
Citations
About This Book
Seeking Symmetry: Finding patterns in human health offers a guide through the overwhelming mass of data generated by contemporary science. Starved for the knowledge that would best help us stay healthy, we are simultaneously glutted with an overload of information about the human body. Amidst ubiquitous talk that patient-centered care and lifestyle changes are the keys to personal health, self-neglect and medical overtreatment nevertheless prevail.
The body is rich with symmetries, many of them unknown to us who live in these bodies. Symmetry-seeking reveals certain patterns for understanding the information we have about the body, patterns whose roots lie in embryonic development and in evolution.
- The book's exploration will guide readers through the parts of their own bodies and introduce tangible, visible examples of symmetry, not only right and left but up and down, male and female, inside and out, as well as symmetries between humans and other species.
- It presents the symmetries of the body's internal structures that, despite their complexity, are nevertheless simple to understand when viewed with an eye for pattern.
- Through both words and images, this book will illustrate the most foundational of the principles, structures, and processes that decide how bodies function.
- A core purpose of the book is to present this knowledge through a lens that makes the information meaningful, by modeling the habit of symmetry-seeking.
Frequently asked questions
At the moment all of our mobile-responsive ePub books are available to download via the app. Most of our PDFs are also available to download and we're working on making the final remaining ones downloadable now. Learn more here.
Both plans give you full access to the library and all of Perlegoâs features. The only differences are the price and subscription period: With the annual plan youâll save around 30% compared to 12 months on the monthly plan.
We are an online textbook subscription service, where you can get access to an entire online library for less than the price of a single book per month. With over 1 million books across 1000+ topics, weâve got you covered! Learn more here.
Look out for the read-aloud symbol on your next book to see if you can listen to it. The read-aloud tool reads text aloud for you, highlighting the text as it is being read. You can pause it, speed it up and slow it down. Learn more here.
Yes, you can access Seeking Symmetry by Niall Galloway in PDF and/or ePUB format, as well as other popular books in Biological Sciences & Biology. We have over one million books available in our catalogue for you to explore.
Information
1
Human architecture
One hundred years ago, the famous Scottish biologist DâArcy Thompson spotted the physics of living things: âCell and tissue, shell and bone, leaf and flower are so many portions of matter, and it is in obedience to the laws of physics that their particles have been moved, moulded and conformedâ. A great observer of nature, Thompson was intrigued at how often âthe forms of living things and the parts of living things can be explained by physical considerations and to realize that in general no organic forms exist save such as are in conformity with physical and mathematical lawsâ.1 Our human bodies are one of those living things, also âso many portions of matterâ, and our living particles have been moved, molded, and conformed in accordance with those same general physical laws. In his recent book Design in Nature, Adrian Bejan arrives at similar conclusions about an even broader range of organisms and systems. Exploring familiar forms such as trees and rivers, vascular branching, and respiratory airways as well as electricity in circuit boards, he proposes that the shape shared among all these forms arises from their common purpose â that they are all systems evolved to promote ever more efficient forward flow.2
What Bejan calls âflowâ is a shared feature of many natural systems, from biology to geography, all of which involve movement. In a storm, thunder and lightning reflect the sound and sight of electrical discharge flowing from high-energy clouds to less-charged clouds. Flow always favors the path of least resistance, so whatever needs to move takes the easiest way it can find. Electrical charge hops within and between clouds, building potential for discharge until, in an instant, one big streak bolts to the nearest high ground, cascading down to strike a tree or rooftop. The forked lightening is instantaneous and fleeting, but its pattern mirrors that of other familiar forms, whether tree roots, river estuaries, or small veins that come together to form larger ones (venous arcades). The pattern repeats again and again: many and smaller unite to become fewer and larger and, in the process, weaker and slower become stronger and faster.
What is flowing doesnât matter nearly so much as the fact of flow, according to Bejan, who tells us that these naturally made fractal structures exhibit shared shape and form because of their shared function and purpose, always promoting forward movement. The physical laws that govern the patterns of flow will apply equally to all that is flowing, and the field will be shaped according to those shared efficiencies and constraints. Whether electrical charge, water, blood, or air, if the system is about flow, the physical laws will define and channel that flow in familiar and recurring patterns, which reflect optimal streaming.
Just as Bejan makes the case for patterns of flow, so it is for patterns of growth. When DâArcy Thompson observed the striking patterns of similarity between many animalsâ shells and bones, he posited that they shared common features of form because they shared common laws of growth. Those laws, in turn, emerged from parallel needs for function, which create similar physical constraints and efficiencies. Growing in organisms â like flowing, in the systems Bejan examined â is governed by fixed natural laws. Those rules of growing and flowing all generate similar shapes, which are therefore widely familiar to us all. We shall explore some of what is now known about the rules of growth and discover that, in living systems, not only the rules but also the ancient tools for growth are shared and common to all.

Figure 1.1
Selective renal arteriography â left kidney. This X-ray image of the kidney is obtained by injecting contrast medium into the renal artery to display the vessels that carry blood from the heart to the kidney tissue. The vascular bed has the familiar form of a tree, with one or more trunks, dividing into a few major branches, more smaller branches and many tiny twigs. Another tree of vessels collects the blood from the tissues as tiny vessels called venules that join together to form larger veins, and in turn, large venous trunks that drain the blood back to the heart.
The surgeon must often raise a flap of adjacent skin and turn it to a new position, just as the tailor might cut cloth and sew it in place to cover a contiguous part. Easier for the tailor, because fabric needs no blood supply; skin, on the other hand, must be nourished. It depends on neighboring areas of skin, which must be the bridge to adjacent blood vessels that can keep the skin tissue alive. In surgery, experience teaches us that skin flaps depend critically on the base of skin they attach to, and the geometry is simple: to survive, the skin flap must be no longer than its base is wide. The wider the base, the larger the skin flap can be. But the flap must never be longer than the width of its base, else some of the skin at the tip will perish and die. The foundation is key. The ideal flap is square-shaped, attached along one side but free on the other three edges. And for living skin to survive, the skin flap must never be longer than it is wide, so only a square-shaped flap provides the ideal ratio â maximum area for coverage but without risk of skin breakdown.
These flaps of living skin that we sew together during surgery follow a predictable model, on a principle that applies not just for repairs in adult patients but, viewed through the eyes of symmetry-seeking, for growth during early life, whether in an embryo, fetus, or child. Whereas the skin is flat, the structures the embryo is growing are fully three-dimensional, but the mathematical principle is the same: the dimensions of the base will support only a given amount of living tissue and no more. So, just as living skin needs to attach to a base of a length no larger than itself, to survive, newly forming body parts and vital organs each need a base with a larger volume than themselves as a foundation of support from which to grow. We grow, then, from a base of big parts out to smaller parts, which comes as no surprise: we grow our legs, then our feet, and then our toes. A careful look at the patterns of that growth, in keeping with Thompsonâs findings, does reveal a surprise, however: a constantly predictable set of proportions in those dimensions.

Figure 1.2
Skin flap. In raising a flap of living skin, the length must not exceed the width of the base or the tip of the flap will die.
Forearm, hand, and finger proportions: the golden ratio
When you look at your open hand, you can compare the distance from the tip of the middle finger to its base where the finger joins the palm. You will see that the ratio of lengths, tip of the finger to the distal crease (behind the knuckle nearest the tip), and distal crease to the base, is the same as the ratio of the whole finger to the length of the palm, measured to the crease at the wrist.
The length of the whole hand is, in turn, the same ratio to the length of the forearm, from the crease of the wrist to the crease of the elbow. Though we are all different sizes, some shorter and others longer, making absolute lengths less useful, the proportions are reliably consistent, like Da Vinciâs Vitruvian Man. You can also see that the part nearer the base of a limb is always a little thicker and more substantial, because it is the foundation upon which the next section is grounded. Like a cone, the wider base supports the lesser, more distal segments.
When we compare two numbers, such as these measurements, we call that relationship a ratio. But when we compare two ratios, we call that relationship a proportion, such as a part of the finger is to the whole as the whole finger is to palm, or finger is to palm as whole hand is to forearm. And this proportion we see in our limbs and digits is called âgoldenâ, for its qualities are not only exquisite and astonishing but also unique and, at the same time, universally present â not only in our human bodies but throughout the living world. The âgolden sectionâ divides a line such that, through ever-larger body parts, the length of the shorter portion is to the length of the longer as the longer part is to the whole.
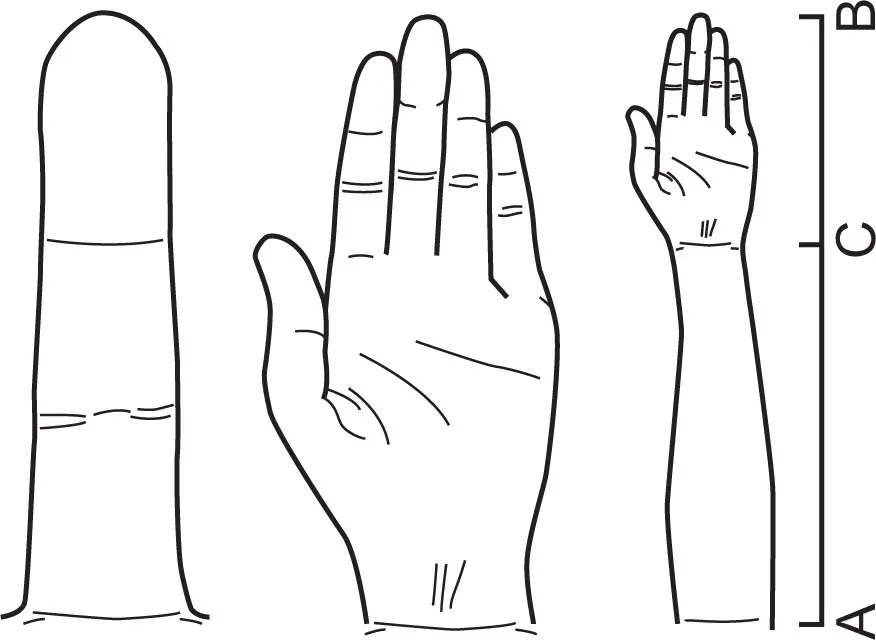
Figure 1.3
Golden ratio proportions, finger to hand and hand to forearm.

Figure 1.4
Golden section. The five-pointed star is made exclusively of lengths of golden section proportions.

Figure 1.5
Golden section in the face, teeth and EKG.
Another way to define the golden section would be the division of a line into two segments such that the whole line is to the longer length as the longer is to the shorter. This sounds so simple, but it is also profound. The golden section could equally be called the growth section, because this proportion is the hallmark of living systems, even our own facial proportions. Look at your face in a mirror and seek out the golden section. It is in the form of your face and the size of your teeth.
We are all different in detail, but broadly similar in form. Human faces evolved to be similar enough to other humans to be able to tell humans from beasts but different enough to tell one human from another. Hundreds of millions of faces on passport photographs and each one just a little different from the next: like natureâs specimens, endless variations on modest themes. And not just faces: look at your torso or your own standing figure, simultaneously yours alone, but also like the rest. And somehow, we are all made in golden section proportions.

Figure 1.6
The umbilicus in this figure marks the golden section.
Tensegrity
Relatively little is known about the forces that guide molecules to self-assemble for creating proteins, cells, and tissues, but it is clear that all living structures are constructed using the same basic architecture. Just as the human body is a balance made of compression-bearing bones and flexible or tension-bearing muscles, tendons, and fascia, so all living systems at all levels of scale are made the same way: stiffer struts balance with stretchy strings that hold our structures together, even at the level of our individual proteins and single cells.
In large-scale construction projects, engineers have found ways to design lightweight but sturdy structures that mimic nature, seeming to hold themselves together in midair as if by magic. This kind of architecture, called tensegrity, allows complementary opposite forces to equilibrate throughout the whole and stabilize the shape and form. We see this pattern in some very modern buildings and bridges, which rely on the strangely simple structure of like parts linked together in set proportions with others to counterbalance them.
The term âtensegrityâ was first coined by architect and inventor Richard Buckminster Fuller, combining the qualities of âtensionâ and âintegrityâ to describe structures that comprise a balance of tensioning and compressive forces distributed and balanced within a structure. Always acting together as complementary opposites, tension elements are arranged in fixed proportion with compression elements. All the structural components are already prestressed in tension or compression, and mechanical stability and strength come from the way the entire structure distributes and balances force and energy.
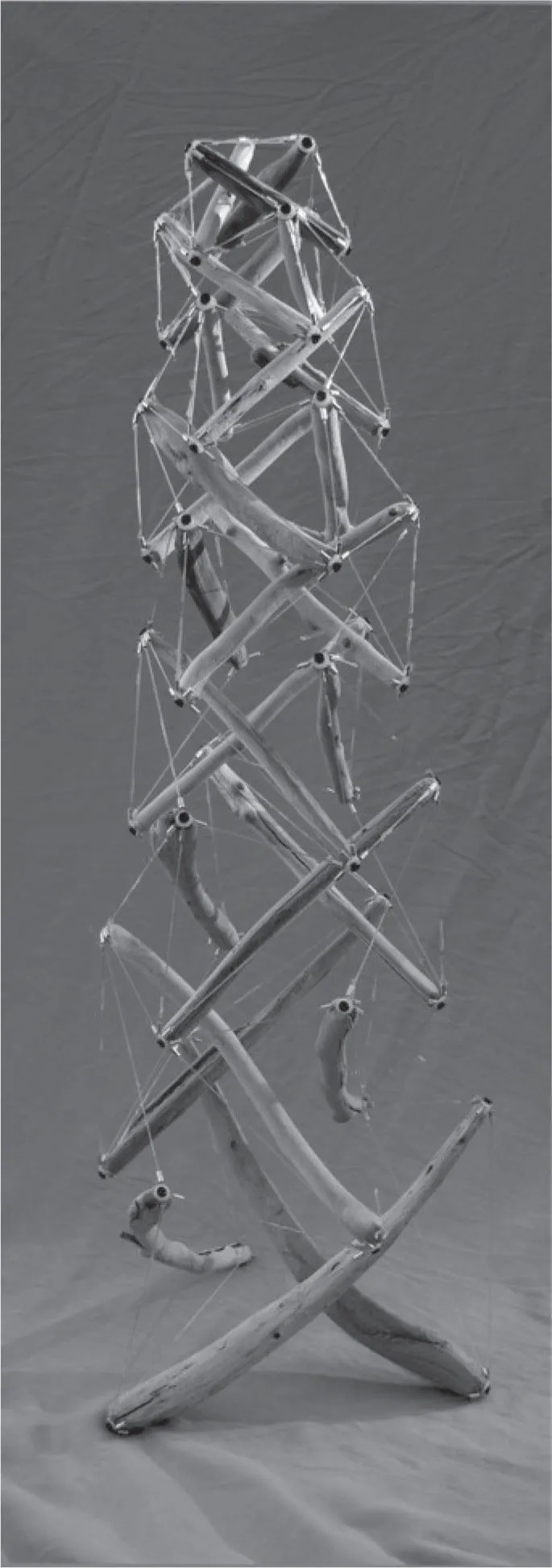
Figure 1.7
Tensegrity architecture: a driftwood tower constructed by Bruce Hamilton.
Traditional man-made walls or bridges gain their stability from the continuous compression of stacking one on one, relying on the constant pull of gravity, but tensegrity structures distribute tension continuously throughout the whole. In tensegrity architecture, increasing tension in one place simultaneously alters tension throughout the entire structure, even in elements on the opposite side. In this way, the construction maintains structural integrity by balancing the global increase in tension with a symmetrical increase in compression, in certain other members spaced throughout the structure.
In bridges or domes, tensegrity structures bring a maximum amount of strength for the least mass of building material. This same economy, and its consequent adaptability and flexibility, also makes tensegrity architecture the perfect choice for building living systems, where it is called biotensegrity. This concept was first introduced by orthopedic surgeon, Dr Stephen Levin, who has pioneered the ideas of tensegrity architecture and led the way for others to explore these ideas in biology and medicine. This method of construction scales from the macroscopic level â the whole body and larger â down to the microscopic and smaller: cells and molecules, including proteins and DNA. All these systems within systems stabilize themselves through the principles of tensegrity architecture.
In his recent book Biotensegrity: The Structural Basis of Life, Graham Scarr describes this living architecture.3 This architecture applies at all size scales, progressing from atoms to the basic building blocks of protein molecules â including the regulatory bases of DNA â and on to individual cells, tissues, organs, and whole bodies. At the molecular level, electrical charges provide the pull and push of attracting and repelling, manifesting the physical laws that shape protein, starch, and lipid molecules. In cell walls, complex chains of fatty acids align like mini-magnets according to physical laws of the hydrophilic and hydrophobic, self-assembling cell-wall structure in ways similar to soap and water making bubbles, or ice and water vapor making snowflakes.
Cell structure
Ideas about cell structure have changed over the years, as more has been discovered about cellular and subcellular architecture. When I was at medical school, doctors imagined the human cell to be like a tiny balloon filled with jelly, its outer membrane like a little bag that enclosed its contents. Cells were known to have an internal framework, or cytoskeleton, composed of microfilaments and microtubules, but their functions were very poorly understood. Another mystery was how single cells of the body moved or might behave when placed on different surfaces. In a cell culture they would usually spread out and flatten on a glass surface or in the plastic culture dish.
In 1980, Albert K. Harris demonstrated that when grown on a thin, flexible sheet of silicon rubber, cells would contract and become more spherical, even puckering up the silicon into folds. H...
Table of contents
- Title Page
- Copyright
- Contents
- Foreword by Stephen Levin
- Foreword by John Sharkey
- Introduction
- Chapter 1: Human architecture
- Chapter 2: Heads over tails: looking for patterns
- Chapter 3: Not just parts: look at the whole body
- Chapter 4: Hormones: the common currency across species
- Chapter 5: Symmetry reveals shared targets and drug actions
- Chapter 6: Fueling the fire: downstream drift
- Chapter 7: Animal farming and disease
- Chapter 8: Diseases of food: looking beyond medicine, to causes
- Chapter 9: Using our brains
- Chapter 10: Confluence over conflict
- Chapter 11: Conclusion: using the symmetry-seeking toolkit
- Index