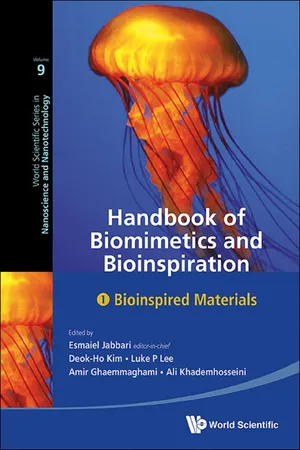
Handbook Of Biomimetics And Bioinspiration: Biologically-driven Engineering Of Materials, Processes, Devices, And Systems (In 3 Volumes)
Biologically-Driven Engineering of Materials, Processes, Devices, and Systems(In 3 Volumes)
Esmaiel Jabbari, Deok-Ho Kim, Luke P Lee, Amir Ghaemmaghami, Ali Khademhosseini
- 484 Seiten
- English
- ePUB (handyfreundlich)
- Über iOS und Android verfügbar
Handbook Of Biomimetics And Bioinspiration: Biologically-driven Engineering Of Materials, Processes, Devices, And Systems (In 3 Volumes)
Biologically-Driven Engineering of Materials, Processes, Devices, and Systems(In 3 Volumes)
Esmaiel Jabbari, Deok-Ho Kim, Luke P Lee, Amir Ghaemmaghami, Ali Khademhosseini
Über dieses Buch
Global warming, pollution, food and water shortage, cyberspace insecurity, over-population, land erosion, and an overburdened health care system are major issues facing the human race and our planet. These challenges have presented a mandate to develop “natural” or “green” technologies using nature and the living system as a guide to rationally design processes, devices, and systems. This approach has given rise to a new paradigm, one in which innovation goes hand-in-hand with less waste, less pollution, and less invasiveness to life on earth. Bioinspiration has also led to the development of technologies that mimic the hierarchical complexity of biological systems, leading to novel highly efficient, more reliable multifunctional materials, devices, and systems that can perform multiple tasks at one time. This multi-volume handbook focuses on the application of biomimetics and bioinspiration in medicine and engineering to produce miniaturized multi-functional materials, devices, and systems to perform complex tasks. Our understanding of complex biological systems at different length scales has increased dramatically as our ability to observe nature has expanded from macro to molecular scale, leading to the rational biologically-driven design to find solution to technological problems in medicine and engineering.
The following three-volume set covers the fields of bioinspired materials, electromechanical systems developed from concepts inspired by nature, and tissue models respectively.
-->
- Volume 1: Bioinspired Materials
- Volume 2: Electromechanical Systems
- Volume 3: Tissue Models
-->
The first volume focuses on the rational design of nano- and micro-structured hierarchical materials inspired by the relevant characteristics in living systems, such as the self-cleaning ability of lotus leaves and cicadas' wings; the superior walking ability of water striders; the anti-fogging function of mosquitoes' eyes; the water-collecting ability of Namib Desert Beetles and spider silk; the high adhesivity of geckos' feet and rose petals; the high adhesivity of mussels in wet aquatic environments; the anisotropic wetting of butterflies' wings; the anti-reflection capabilities of cicadas' wings; the self-cleaning functionality of fish scales; shape anisotropy of intracellular particles; the dielectric properties of muscles; the light spectral characteristics of plant leaves; the regeneration and self-healing ability of earthworms; the self-repairing ability of lotus leaves; the broadband reflectivity of moths' eyes; the multivalent binding, self-assembly and responsiveness of cellular systems; the biomineral formation in bacteria, plants, invertebrates, and vertebrates; the multi-layer structure of skin; the organization of tissue fibers; DNA structures with metal-mediated artificial base pairs; and the anisotropic microstructure of jellyfish mesogloea. In this volume, sensor and microfluidic technologies combined with surface patterning are explored for the diagnosis and monitoring of diseases. The high throughput combinatorial testing of biomaterials in regenerative medicine is also covered.
The second volume presents nature-oriented studies and developments in the field of electromechanical devices and systems. These include actuators and robots based on the movement of muscles, algal antenna and photoreception; the non-imaging light sensing system of sea stars; the optical system of insect ocellus; smart nanochannels and pumps in cell membranes; neuromuscular and sensory devices that mimic the architecture of peripheral nervous system; olfaction-based odor sensing; cilia-mimetic microfluidic systems; the infrared sensory system of pyrophilous insects; ecologically inspired multizone temperature control systems; cochlea and surface acoustic wave resonators; crickets' cercal system and flow sensing abilities; locusts' wings and flapping micro air vehicles; the visual motion sensing of flying insects; hearing aid devices based on the human cochlea; the geometric perception of tortoises and pigeons; the organic matter sensing capability of cats and dogs; and the silent flight of rats.
The third volume features engineered models of biological tissues. These include engineered matrices to mimic cancer stem cell niches; in vitro models for bone regeneration; models of muscle tissue that enable the study of cardiac infarction and myopathy; 3D models for the differentiation of embryonic stem cells; bioreactors for in vitro cultivation of mammalian cells; human lung, liver and heart tissue models; topographically-defined cell culture models; ECM mimetic tissue printing; biomimetic constructs for regeneration of soft tissues; and engineered constructs for the regeneration of musculoskeletal and corneal tissue.
This three-volume set is a must-have for anyone keen to understand the complexity of biological systems and how that complexity can be mimicked to engineer novel materials, devices and systems to solve pressing technological challenges of the twenty-first century.
Key Features:
- The only handbook that covers all aspects of biomimetics and bioinspiration, including materials, mechanics, signaling and informatics
- Contains 248 colored figures
Contents:
- Bioinspired Materials:
- Bioinspired Fabrication of Nanostructures from Tissue Slices (Kyle Alberti and Qiaobing Xu)
- Bioinspired Artificial Muscles Based on Dielectric Elastomers (Federico Carpi)
- Bioinspired Engineering of Multifunctional Devices (Ryan M Pearson, Ja Hye Myung and Seungpyo Hong)
- Bioinspired Self-Cleaning Antireflection Coatings (Khalid Askar, Blayne M Phillips, Bin Jiang and Peng Jiang)
- Anisotropic Biomimetic Particle Delivery Systems (Stephen C Balmert and Steven R Little)
- Biomimetic Approaches to Peripheral Neuroprosthetic Interfaces (Shawn M Dirk, Patrick Lin, Stephen Buerger, Kirsten N Cicotte, Gregory P Reece and Elizabeth Hedberg-Dirk)
- Biomimetic Superhydrophobic Surfaces (Mariana B Oliveira and João F Mano)
- Clinical Applications of Bioinspired Artificial Dermis (Katsuya Kawai, Naoki Morimoto and Shigehiko Suzuki)
- Contributions of Collagen and Elastin to Mechanobiology of Arteries (Yanhang Zhang and Ming-Jay Chow)
- DNA Inspired Self-Assembled Metal Arrays (Yusuke Takezawa and Mitsuhiko Shionoya)
- Jellyfish Inspired Hybrid Hygrogels (Huiliang Wang)
- Mussel-Inspired Adhesive Biomaterials (Kyuri Kim, Seonki Hong and Haeshin Lee)
- Biomimetic Protamine-Templated Silicification (Yanjun Jiang, Lei Zhang, Jiafu Shi, Jian Li, Dong Yang and Zhongyi Jiang)
- Bionic Materials Based on Spectral Characteristics of Plant Leaves (Biru Hu, Zhiming Liu, Yunqiu Li and Wenjian Wu)
- Bioinspired Design of Super-Antiwetting Interfaces (Dongliang Tian and Lei Jiang)
- Bioinspired Self-Healing Coatings (Xu Wang, Yang Li and Junqi Sun)
- Electromechanical Systems:
- Bioinspired Design of Peripheral Nerve Devices (Sameer B Shah)
- Bioinspired Artificial Muscles and Robots (Il-Kwon Oh and Choonghee Jo)
- Biomimetic Materials and Multivariate Approach to Odor Sensing (Takamichi Nakamoto and Bartosz Wyszynski)
- Biomimetic Cilia (Jae-Hyun Chung, Tae-Rin Lee and Wing Kam Liu)
- Infrared Sensors Inspired by Pyrophilous Insects (Herbert Bousack, Helmut Soltner, David Klocke and Helmut Schmitz)
- Insect Inspired Visual Motion Sensing and Flying Robots (Thibaut Raharijaona, Lubin Kerhuel, Julien Serres, Frédéric Roubieu, Fabien Expert, Stéphane Viollet, Franck Ruffier and Nicolas Franceschini)
- Insect-Inspired Micro Air Vehicles (Rajeev Kumar, Ryan Randall, Dima Silin and Sergey V Shkarayev)
- Microfabricated Auditory System Mimicking Human Cochlea (Hongsoo Choi, Jeong Hun Jang and Won Joon Song)
- Olfactory and Geomagnetic Perception Inspired Bionic Sensing (Yahui Man, Weisong Pan, Yan Liu, Xianli Du and Wenjian Wu)
- Owl Inspired Silent Flight (Thomas Bachmann and Andrea Winzen)
- The Cochlea, Surface Acoustic Waves, and Resonance (Andrew Bell)
- Bioinspired Smart Nanochannels (Wei Guo and Lei Jiang)
- Bioinspired Non-Imaging Optics (Jaeyoun Kim)
- Biomimetic Multizone Temperature Control (Andrés Pantoja, Nicanor Quijano and Germán Obando)
- Hair-Based Flow-Sensing Inspired by the Cricket Cercal System (G J M Krijnen, H Droogendijk, T Steinmann, A Dagamseh, R K Jaganatharaja and J Casas)
- Mechanics-Related Bioinspired Optimization (Xiang Feng, Francis C M Lau and Huiqun Yu)
- Tissue Models:
- Bioinspired Cell Culture Bioreactors (Matteo Moretti, Chiara Arrigoni, Arianna Lovati and Giuseppe Talò)
- Bioinspired Materials for Bone Regenerative Engineering (Roshan James, Meng Deng, Sangamesh G Kumbar and Cato T Laurencin)
- Bioinspired Muscle Tissue Devices (Toshinori Fujie, Serge Ostrovidov, Samad Ahadian, S Prakash Parthiban, Ali Khademhosseini and Hirokazu Kaji)
- Biomimetic 3D Tissue Printing (Falguni Pati, Joydip Kundu, Jin-Hyung Shim and Dong-Woo Cho)
- Biomimetic Human Lung Models (Helen C Harrington, Paul A Cato and Amir M Ghaemmaghami)
- Biomimetic Materials for Cardiac Regeneration (Alexander J Hodge, Petra Kerscher, David A Dunn and Elizabeth A Lipke)
- Biomimetic Regeneration of Corneal Tissue (Li Buay Koh, Kimberley Merrett, Rodolfo Adrian Elizondo and May Griffith)
- Biomimetic Scaffolds for Musculoskeletal Tissue Engineering (Brandon W Engebretson and Vassilios I Sikavitsas)
- Biomimetic Regeneration of Soft Tissues (Mason J Burger, Tajila Mullahkhel, Ian C McPhee, Benjie R Pease, Michael P H Lau and Leonard F Pease III)
- Bioinspired Nanotopographically-Defined Cell Culture Models (Julie Antetomaso, Alex Jiao, Krystal Gauthier-Bell and Deok-Ho Kim)
- Bioinspired Liver Tissue Engineering (Astrid Herrero, Sabine Gerbal-Chaloin and Martine Daujat-Chavanieu)
- Bioinspired 3D Scaffold for Inducing Differentiation of Embryonic Stem Cells (Sha Jin, Xiuli Wang, Weiwei Wang and Kaiming Ye)
- Bioinspired Engineered Matrix to Regulate Cancer Stem Cell Niche (Esmaiel Jabbari)
Readership: Undergraduate and graduate students studying bioengineering, and researchers, engineers, chemists, biologists, physicists, material scientists and physicians.
Häufig gestellte Fragen
Information
Chapter 1
BIOINSPIRED FABRICATION OF NANOSTRUCTURES FROM TISSUE SLICES
Department of Biomedical Engineering, Tufts University,
4 Colby St., Medford, MA 02155
*[email protected]
1.Introduction
2.Tissue Processing and Skiving
2.1.Decellularizing
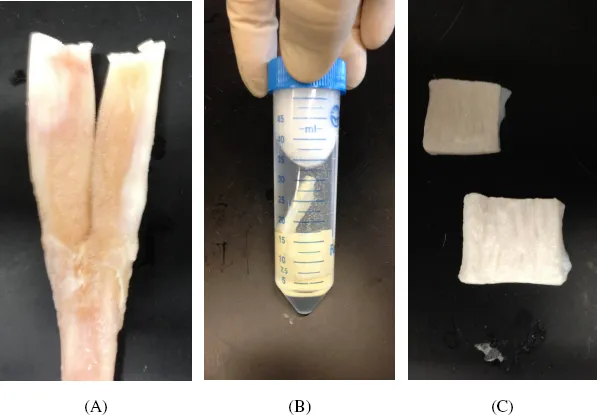
2.2.Sectioning tools
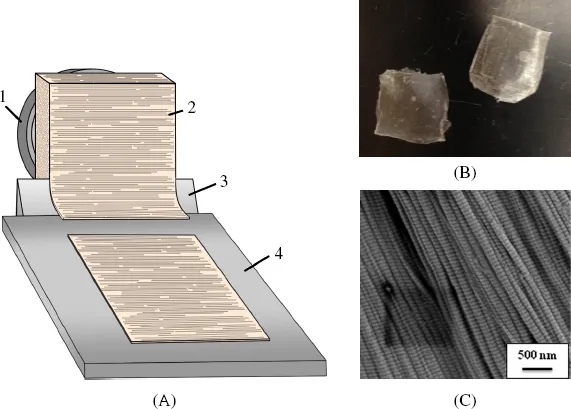
Inhaltsverzeichnis
- Cover Page
- Title
- Copyright
- Preface
- Contents
- About the Editors
- About the Contributors
- 1. Bioinspired Fabrication of Nanostructures from Tissue Slices
- 2. Bioinspired Artificial Muscles Based on Dielectric Elastomers
- 3. Bioinspired Engineering of Multifunctional Devices
- 4. Bioinspired Self-Cleaning Antireflection Coatings
- 5. Anisotropic Biomimetic Particle Delivery Systems
- 6. Biomimetic Approaches to Peripheral Neuroprosthetic Interfaces
- 7. Biomimetic Superhydrophobic Surfaces
- 8. Clinical Applications of Bioinspired Artificial Dermis
- 9. Contributions of Collagen and Elastin to Mechanobiology of Arteries
- 10. DNA Inspired Self-Assembled Metal Arrays
- 11. Jellyfish Inspired Hybrid Hygrogels
- 12. Mussel-Inspired Adhesive Biomaterials
- 13. Biomimetic Protamine-Templated Silicification
- 14. Bionic Materials Based on Spectral Characteristics of Plant Leaves
- 15. Bioinspired Design of Super-Antiwetting Interfaces
- 16. Bioinspired Self-Healing Coatings
- Index
- Cover Page 2
- Title 2
- Copyright 2
- Preface 2
- Contents 2
- About the Editors 2
- About the Contributors 2
- 17. Bioinspired Design of Peripheral Nerve Devices
- 18. Bioinspired Artificial Muscles and Robots
- 19. Biomimetic Materials and Multivariate Approach to Odor Sensing
- 20. Biomimetic Cilia
- 21. Infrared Sensors Inspired by Pyrophilous Insects
- 22. Insect Inspired Visual Motion Sensing and Flying Robots
- 23. Insect-Inspired Micro Air Vehicles
- 24. Microfabricated Auditory System Mimicking Human Cochlea
- 25. Olfactory and Geomagnetic Perception Inspired Bionic Sensing
- 26. Owl Inspired Silent Flight
- 27. The Cochlea, Surface Acoustic Waves, and Resonance
- 28. Bioinspired Smart Nanochannels
- 29. Bioinspired Non-Imaging Optics
- 30. Biomimetic Multizone Temperature Control
- 31. Hair-Based Flow-Sensing Inspired by the Cricket Cercal System
- 32. Mechanics-Related Bioinspired Optimization
- Index
- Cover Page 3
- Title 3
- Copyright 3
- Preface 3
- Contents 3
- About the Editors 3
- About the Contributors 3
- 33. Bioinspired Cell Culture Bioreactors
- 34. Bioinspired Materials for Bone Regenerative Engineering
- 35. Bioinspired Muscle Tissue Devices
- 36. Biomimetic 3D Tissue Printing
- 37. Biomimetic Human Lung Models
- 38. Biomimetic Materials for Cardiac Regeneration
- 39. Biomimetic Regeneration of Corneal Tissue
- 40. Biomimetic Scaffolds for Musculoskeletal Tissue Engineering
- 41. Biomimetic Regeneration of Soft Tissues
- 42. Bioinspired Nanotopographically-Defined Cell Culture Models
- 43. Bioinspired Liver Tissue Engineering
- 44. Bioinspired 3D Scaffold for Inducing Differentiation of Embryonic Stem Cells
- 45. Bioinspired Engineered Matrix to Regulate Cancer Stem Cell Niche
- Index