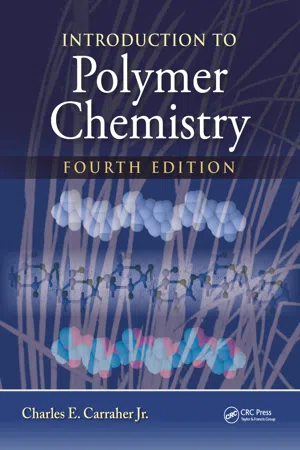
eBook - ePub
Introduction to Polymer Chemistry
Charles E. Carraher Jr.
This is a test
- 560 páginas
- English
- ePUB (apto para móviles)
- Disponible en iOS y Android
eBook - ePub
Introduction to Polymer Chemistry
Charles E. Carraher Jr.
Detalles del libro
Vista previa del libro
Índice
Citas
Información del libro
Introduction to Polymer Chemistry provides undergraduate students with a much-needed, well-rounded presentation of the principles and applications of natural, synthetic, inorganic, and organic polymers. With an emphasis on the environment and green chemistry and materials, this fourth edition continues to provide detailed coverage of natural and synthetic giant molecules, inorganic and organic polymers, elastomers, adhesives, coatings, fibers, plastics, blends, caulks, composites, and ceramics. Building on undergraduate work in foundational courses, the text fulfills the American Chemical Society Committee on Professional Training (ACS CPT) in-depth course requirement
Preguntas frecuentes
¿Cómo cancelo mi suscripción?
¿Cómo descargo los libros?
Por el momento, todos nuestros libros ePub adaptables a dispositivos móviles se pueden descargar a través de la aplicación. La mayor parte de nuestros PDF también se puede descargar y ya estamos trabajando para que el resto también sea descargable. Obtén más información aquí.
¿En qué se diferencian los planes de precios?
Ambos planes te permiten acceder por completo a la biblioteca y a todas las funciones de Perlego. Las únicas diferencias son el precio y el período de suscripción: con el plan anual ahorrarás en torno a un 30 % en comparación con 12 meses de un plan mensual.
¿Qué es Perlego?
Somos un servicio de suscripción de libros de texto en línea que te permite acceder a toda una biblioteca en línea por menos de lo que cuesta un libro al mes. Con más de un millón de libros sobre más de 1000 categorías, ¡tenemos todo lo que necesitas! Obtén más información aquí.
¿Perlego ofrece la función de texto a voz?
Busca el símbolo de lectura en voz alta en tu próximo libro para ver si puedes escucharlo. La herramienta de lectura en voz alta lee el texto en voz alta por ti, resaltando el texto a medida que se lee. Puedes pausarla, acelerarla y ralentizarla. Obtén más información aquí.
¿Es Introduction to Polymer Chemistry un PDF/ePUB en línea?
Sí, puedes acceder a Introduction to Polymer Chemistry de Charles E. Carraher Jr. en formato PDF o ePUB, así como a otros libros populares de Physical Sciences y Chemistry. Tenemos más de un millón de libros disponibles en nuestro catálogo para que explores.
Información
Introduction to Polymers 1
1.1 HISTORY OF POLYMERS
Since most materials are polymeric and most of the recent advances in science and technology involve polymers, some have called this the polymer age. Actually, we have always lived in a polymer age. The ancient Greeks classified all matter as animal, vegetable, and mineral. Minerals were emphasized by the alchemists, but medieval artisans emphasized animal and vegetable matter. All are largely polymeric and are important to life as we know it. Most chemists, biochemists, and chemical engineers are now involved in some phase of polymer science or technology.
The word “polymer” is derived from the Greek poly and meros, meaning many and parts, respectively. Some scientists prefer to use the word macromolecule, or large molecule, instead of polymer. Others maintain that naturally occurring polymers, or biopolymers, and synthetic polymers should be studied in different courses. Others name these large molecules simply “giant molecules.” However, the same principles apply to all polymers. If one discounts the end uses, the differences between all polymers, including plastics, fibers, and elastomers, or rubbers, and natural and synthetic, are determined primarily by the intermolecular and intramolecular forces between the molecules and within the individual molecule, respectively, and by the functional groups present, and most of all, by their size allowing an accumulation of these forces.
In addition to being the basis of life itself, protein is used as a source of amino acids and energy. The ancients degraded or depolymerized the protein in meat by aging and cooking, and they denatured egg albumin by heating or adding vinegar to the eggs. Early humans learned how to process, dye, and weave the natural proteinaceous fibers of wool and silk and the carbohydrate fibers from flax and cotton. Early South American civilizations, such as the Aztecs, used natural rubber (Hevea brasiliensis) for making elastic articles and for waterproofing fabrics.
There has always been an abundance of natural fibers and elastomers but few plastics. Of course, early humans employed a crude plastic art in tanning the protein in animal skins to make leather and through heating created somewhat “plastic” tortoise shells. They also used naturally occurring tars as caulking materials and extracted shellac from the excrement of small coccid insects (Coccus lacca).
Until Wohler synthesized urea from inorganic compounds in 1828, there had been little progress in organic chemistry since the alchemists emphasized the transmutation of base metals to gold and believed in a vital force theory. Despite this essential breakthrough, little progress was made in understanding organic compounds until the 1850s when Kekule developed the presently accepted technique for writing structural formulas. However, polymer scientists displayed a talent for making empirical discoveries before the science was developed.
The original connection between rubber and sulfur is often attributed to Nathaniel Hayward, an American. He is reported to have dusted rubber with sulfur powder and exposed the dusted rubber to sunlight as a way to remove the rubber’s stickiness. Hayward patented his discovery. He turned over his discovery to Charles Goodyear who transformed the connection between sulfur and rubber into the rubber industry.
Charles Goodyear grew up in poverty. He was a Connecticut Yankee born in 1800. He began to work in his father’s farm implements business. Later, he moved to Philadelphia where he opened a retail hardware store that soon went bankrupt. Charles then turned to being an inventor. As a child, he had noticed the magic material that formed a rubber bottle he had found. He visited the Roxbury India Rubber Company to try and interest them in his efforts to improve the properties of rubber. They assured him that there was no need to do so.
He started his experiments with a malodorous gum from South America in debtor’s prison. In a small cottage on the grounds of the prison, he blended the gum, the raw rubber called Hevea rubber, with anything he could find—ink, soup, castor oil, etc. While rubber-based products were available, they were either sticky or became sticky in the summer’s heat. He found that treatment of the raw rubber with nitric acid allowed the material to resist heat and not to adhere to itself. This success attracted backers who helped form a rubber company. After some effort, he obtained a contract to supply the U.S. Post Office with 150 rubber mailbags. He made the bags and stored them in a hot room while he and his family were away. When they returned, they found the bags in a corner of the room, joined together as a single mass. The nitric acid treatment was sufficient to prevent surface stickiness, but the internal rubber remained tacky and susceptible to heat.
While doing experiments in 1839 at a Massachusetts rubber factory, Charles accidentally dropped a lump of rubber mixed with sulfur on the hot stove. The rubber did not melt but rather charred. He had discovered vulcanization, the secret that was to make rubber a commercial success. While he had discovered vulcanization, it would take several years of ongoing experimentation before the process was really commercially useful. During this time, he and his family were near penniless. While he patented the process, the process was too easily copied and pirated so that he was not able to fully profit from his invention and years of hard work. Even so, he was able to develop a number of items.
Charles Goodyear, and his brother Nelson, transformed natural rubber, Hevea rubber, from a heat “softenable” thermoplastic to a less-heat-sensitive product through the creation of cross-links between the individual polyisoprene chainlike molecules using sulfur as the cross-linking agent. Thermoplastics are 2D molecules that may be softened by heat. Thermosets are materials that are 3D networks that cannot be reshaped by heating. Rather than melting, thermosets degrade. As the amount of sulfur was increased, the rubber became harder becoming a hard rubberlike (ebonite) material.
The spring of 1851 found the construction of a remarkable building on the lawns of London’s Hyde Park. The building was designed by a maker of greenhouses so it was not unexpected that it had a “greenhouse look.” This Crystal Palace was to house almost 14,000 exhibitors from all over the world. It was the chance for exhibitors to show their wares. Charles Goodyear, then 50 years old, used this opportunity to show off his over two decades worth of rubber-related products. He decorated his Vulcanite Court with rubber walls, roof, furniture, buttons, toys, carpet, combs, etc. Above it hung a giant six foot rubber raft and assorted balloons. The European public was introduced to the world of new man-made materials.
Within a little more than a decade, Charles Goodyear was dead. Within a year of his death, the American Civil War broke out. The Union military used about $27 million worth of rubber products by 1865 helping launch the American rubber industry.
In 1862, Queen Victoria, while in mourning for her recently departed husband Albert, opened the world’s fair in London. One of the exhibitors was Alexander Parks. He was displeased with the limited colors available for rubber products—generally dull and dark. In his workshop in Birmingham, England, he was working with nitrocellulose, a material made from the treatment of cotton with nitric and sulfuric acids. Nitrocellulose solutions were made from organic liquids such as ethanol and ether. Thin films and coatings were made by simply pouring the nitrocellulose solutions onto the desired item or surface and allowing the solvent to evaporate. He wanted to make solid objects from nitrocellulose. After years of work, he developed a material he called Parkesine from which he made buttons and combs, and in fact many of the items were made of rubber—except that his materials could be brightly colored, clear, or made to shine like mother-of-pearl. At the London world’s fair, he advertised “PATENT PARKESINE of various colours: hard elastic, transparent, opaque, and waterproof.” Even with his work he had not developed a material that could be “worked” or was stable and even with his hype, the material never caught on except within exhibition halls.
About this time, John Wesley Hyatt, a printer from Albany, NY, was seeking a $10,000 prize for anyone who could come up with a material that was a substitute for ivory billiard balls, developed a material that was stable and could be “worked” from shellac and wood pulp. He then turned to nitrocellulose discovering that shredded nitrocellulose could be mixed with camphor, heated under pressure, to produce a tough white mass that retained its shape. This material, dubbed celluloid, could be made into the usual rubberlike products, but also solid pieces like boxes, wipe-clean linen collars, cuffs, and ping-pong balls. Celluloid could also, like the shellac-wood pulp mixture, be worked—cut, drilled, and sawed. But celluloid was flammable and did not stand up well in hot water. The wearers of celluloid dentures truly could have their “teeth curled” when drinking a hot cup of coffee. One of its best qualities was that it could be made to “look like” other materials—it could be dyed to look like marble and swirled to mimic tortoiseshell and mother-of-pearl and even look and feel like ivory. It did not make good billiard balls. One account has billiard balls hitting and exploding like a shot that caused cowboys to draw their guns.
Both cellulose and cellulose nitrate are linear, or 2D, polymers, but the former cannot be softened because of the presence of multitudinous hydrogen bonds between the chainlike molecules. When used as an explosive, the cellulose nitrate is essentially completely nitrated, but the material used by Parks and Hyatt was a dinitrate, still potentially explosive, but less so. Parks added castor oil and Hyatt added camphor to plasticize—reduce the effect of the hydrogen bonding—the cellulose nitrate allowing it some flexibility.
Worldwide, rubber gained in importance with the invention of the air-filled or pneumatic tires by a Scotsman, John Dunlop in 1888. He had a successful veterinarian practice in Belfast. In his off time, he worked to improve the ride of his son’s tricycle. His invention happened at the right time. The automobile was emerging and the air-filled tires offered a gentler ride. Thus, was begun the tire industry.
All of these inventions utilized natural materials as at least one ingredient. After years of work in his chemistry labs in Yonkers, NY, Leo Baekeland in 1907 announced in an American Chemical Society meeting the synthesis of the first truly synthetic polymeric material latter dubbed Bakelite.
Baekeland was born in Belgium in 1863, the son of an illiterate shoe repairman and a maid. He was bright and received, with highest honors, his doctorate at the age of 20. He could have spent the remaining part of his life in academics in Europe, but heading the words of Benjamin Franklin, he sailed to America. In the 1890s, he developed the first photographic paper, called Velox, which could be developed in synthetic light rather than sunlight. George Eastman saw the importance of this discovery and paid Bakeland $750,000 for the rights to use this invention.
It was generally recognized by the leading organic chemists of the nineteenth century that phenol would condense with formaldehyde. Since they did not recognize the concept of functionality, Baeyer, Michael, and Kleeberg produced useless cross-linked goos, gunks, and messes and then returned to their research on reactions of monofunctional reactants. However, by the use of a large excess of phenol, Smith, Luft, and Blumer were able to obtain a hard, but meltable thermoplastic material.
With his $750,000, Baekeland set up a lab next to his home. He then sought to solve the problem of making the hard material made from phenol and formaldehyde soluble. After many failures, he thought about circumventing the problem by placing the reactants in a mold of the desired shape and allowing them to form the intractable solid material. After much effort, he found the conditions under which a hard, clear solid could be made—Bakelite was discovered. Bakelite could be worked, it was resistant to acids and organic liquids, stood up well to heat and electrical charge, and could be dyed to give colorful products. It was used to make bowling balls, phonograph records, telephone housings, gears, and cookware. His materials also made excellent billiard balls. Bakelite also acted as a binder for sawdust, textiles, and paper forming a wide range of composites including Formica laminates, many of which are still used. It was also used as an adhesive giving us plywood.
While there is no evidence that Baekeland recognized what polymers were, he appeared to have a grasp on functionality and how to “use” it to produce thermoplastic materials that could later be converted to thermosets. Through control of the ratio of phenol to formaldehyde, he was able to form a material that was a thermoplastic. He coined the term “A-stage resole resin” to descri...
Índice
- Cover Page
- Title Page
- Copyright Page
- Contents
- Preface
- Acknowledgments
- Author
- How to Study Polymers
- Polymer Nomenclature
- American Chemistry Society Committee on Professional Training Requirements
- Chapter 1 Introduction to Polymers
- Chapter 2 Polymer Structure (Morphology)
- Chapter 3 Molecular Weight of Polymers
- Chapter 4 Naturally Occurring Polymers
- Chapter 5 Step-Reaction Polymerization (Polycondensation Reactions)
- Chapter 6 Ionic Chain Reaction and Complex Coordination Polymerization (Addition Polymerization)
- Chapter 7 Free Radical Chain Polymerization (Addition Polymerization)
- Chapter 8 Copolymerization
- Chapter 9 Organometallic and Metalloid Polymers
- Chapter 10 Inorganic Polymers
- Chapter 11 Reactions of Polymers
- Chapter 12 Testing and Spectrometric Characterization of Polymers
- Chapter 13 Rheology and Physical Tests
- Chapter 14 Additives
- Chapter 15 Synthesis of Reactants and Intermediates for Polymers
- Chapter 16 Polymer Technology
- Appendix A: Structures of Common Polymers
- Appendix B: Symbols and Acronyms
- Appendix C: Comments on Health
- Appendix D: ISO 9000 and 14000
- Appendix E: Web Information
- Index
Estilos de citas para Introduction to Polymer Chemistry
APA 6 Citation
Carraher, C. (2017). Introduction to Polymer Chemistry (4th ed.). CRC Press. Retrieved from https://www.perlego.com/book/1575715/introduction-to-polymer-chemistry-pdf (Original work published 2017)
Chicago Citation
Carraher, Charles. (2017) 2017. Introduction to Polymer Chemistry. 4th ed. CRC Press. https://www.perlego.com/book/1575715/introduction-to-polymer-chemistry-pdf.
Harvard Citation
Carraher, C. (2017) Introduction to Polymer Chemistry. 4th edn. CRC Press. Available at: https://www.perlego.com/book/1575715/introduction-to-polymer-chemistry-pdf (Accessed: 14 October 2022).
MLA 7 Citation
Carraher, Charles. Introduction to Polymer Chemistry. 4th ed. CRC Press, 2017. Web. 14 Oct. 2022.