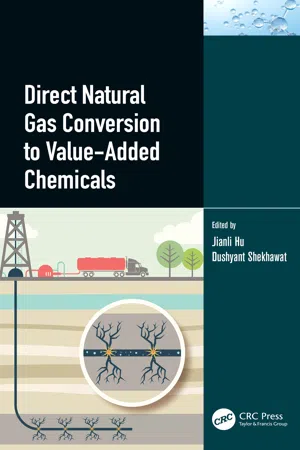
eBook - ePub
Direct Natural Gas Conversion to Value-Added Chemicals
Jianli Hu, Dushyant Shekhawat, Jianli Hu, Dushyant Shekhawat
This is a test
- 454 páginas
- English
- ePUB (apto para móviles)
- Disponible en iOS y Android
eBook - ePub
Direct Natural Gas Conversion to Value-Added Chemicals
Jianli Hu, Dushyant Shekhawat, Jianli Hu, Dushyant Shekhawat
Detalles del libro
Vista previa del libro
Índice
Citas
Información del libro
Direct Natural Gas Conversion to Value-Added Chemicals comprehensively discusses all major aspects of natural gas conversion and introduces a broad spectrum of recent technological developments. Specifically, the book describes heterogeneous and homogeneous catalysis, microwave-assisted conversion, non-thermal plasma conversion, electrochemical conversion, and novel chemical looping conversion approaches.
-
- Provides an excellent benchmark resource for the industry and academics
-
- Appeals to experienced researchers as well as newcomers to the field, despite the variety of contributing authors and the complexity of the material covered
-
- Includes all aspects of direct natural gas conversion: fundamental chemistry, different routes of conversion, catalysts, catalyst deactivation, reaction engineering, novel conversion concepts, thermodynamics, heat and mass transfer issues, system design, and recent research and development
-
- Discusses new developments in natural gas conversion and future challenges and opportunities
This book is as an excellent resource for advanced students, technology developers, and researchers in chemical engineering, industrial chemistry, and others interested in the conversion of natural gas.
Preguntas frecuentes
¿Cómo cancelo mi suscripción?
¿Cómo descargo los libros?
Por el momento, todos nuestros libros ePub adaptables a dispositivos móviles se pueden descargar a través de la aplicación. La mayor parte de nuestros PDF también se puede descargar y ya estamos trabajando para que el resto también sea descargable. Obtén más información aquí.
¿En qué se diferencian los planes de precios?
Ambos planes te permiten acceder por completo a la biblioteca y a todas las funciones de Perlego. Las únicas diferencias son el precio y el período de suscripción: con el plan anual ahorrarás en torno a un 30 % en comparación con 12 meses de un plan mensual.
¿Qué es Perlego?
Somos un servicio de suscripción de libros de texto en línea que te permite acceder a toda una biblioteca en línea por menos de lo que cuesta un libro al mes. Con más de un millón de libros sobre más de 1000 categorías, ¡tenemos todo lo que necesitas! Obtén más información aquí.
¿Perlego ofrece la función de texto a voz?
Busca el símbolo de lectura en voz alta en tu próximo libro para ver si puedes escucharlo. La herramienta de lectura en voz alta lee el texto en voz alta por ti, resaltando el texto a medida que se lee. Puedes pausarla, acelerarla y ralentizarla. Obtén más información aquí.
¿Es Direct Natural Gas Conversion to Value-Added Chemicals un PDF/ePUB en línea?
Sí, puedes acceder a Direct Natural Gas Conversion to Value-Added Chemicals de Jianli Hu, Dushyant Shekhawat, Jianli Hu, Dushyant Shekhawat en formato PDF o ePUB, así como a otros libros populares de Ciencias biológicas y Biofísica. Tenemos más de un millón de libros disponibles en nuestro catálogo para que explores.
Información
1 Electrochemical Conversion of Natural Gas to Value Added Chemicals
Wei Wu, Anne Gaffney, and Dong Ding
CONTENTS
1.1 Introduction
1.2 Electrochemical Direct Conversion of Methane
1.2.1 Electrochemical Methane Coupling to Produce Ethylene
1.2.1.1 Electrochemical Oxidative Methane Coupling (EOMC)
1.2.1.2 Electrochemical Nonoxidative Methane Coupling (ENMC)
1.2.2 Electrochemical Methane Dehydro-Aromatization (EDMA)
1.3 Electrochemical Direct Conversion of Ethane
1.3.1 Electrochemical Oxidative Dehydrogenation (EODH)
1.3.2 Electrochemical Nonoxidative Dehydrogenation (ENDH)
1.4 Electrochemical Direct Conversion of Propane
1.5 Challenges and Opportunities
Acknowledgments
References
1.1 Introduction
The shale gas revolution results in an increasingly abundant production of natural gas (NG) and light hydrocarbons, most of which is located in remote areas that making transportation through pipelines less economic attractive. In consequence, conversion of NG into value added chemicals and fuels has become increasingly important for petrochemical industry, which has noticed the opportunities for new business and technologies, i.e., how to efficiently produce value added higher hydrocarbons from light alkanes. Since the top three contents of NG are methane (70~98%), ethane and propane (0~18% in total), NG conversion is commonly treated as conversion of light alkanes (i.e., methane conversion and ethane conversion). Methane can be converted to chemicals and fuels in two ways, either through synthesis gas or directly into C2 hydrocarbons and/or aromatics. Ethane and propane are normally used to produce ethylene and propylene through steam cracking that represents one of the most energy-consuming process in petrochemical industry. Take ethylene production for an example. The ethane steam cracking method is an energy-intensive, nonselective and noncatalytic technology, producing an array of chemicals including hydrogen, ethylene, acetylene, propylene, etc. In addition to the high temperature needed, steam cracking requires high investment in separation and purification equipment (Ren, Patel, and Blok 2006). The ethane steam cracking process consumes 17–21 GJ (specific energy consumption, SEC) of process energy per ton of ethylene, of which 65% is used in high temperature pyrolysis, 15% in fractionation and compression, and 20% in product separation.(Kaiser et al. 1993; Houston 1993; Worrell et al. 2000) Not only is the current steam cracking technology capital and energy intensive, it is a large carbon dioxide producer (1–1.5 t/t of ethylene) (Ramirez-Corredores, Ding, and Gaffney 2019).
However, the current high temperature steam-cracking technology is hard to be replaced due to the maturity of the manufacturing industry, where moderate changes were made over the past 50 years. The energy efficiency was also optimized by reordering of the separation units, improvements in controls, and reactor redesign etc., with reliable operation. In order to reduce both the energy consumption and the CO2 emission in NG conversion, disruptive methods that feature a lower working temperature with higher energy efficiency, instead of simple process optimization, are preferred, because the working temperature and the energy efficiency are critical in NG and NGLs related manufacturing (Gao et al. 2019).
Electrochemistry, which has been in development for centuries, holds great potential in NG conversion and chemical engineering technology. Electrochemical methods are increasingly attractive in converting electricity power into chemical bonds for energy storage and have obvious advantages over traditional thermochemical methods. First, electrochemical methods use electricity as alternative driving force to enable bond-formation steps, making most electrochemical reactions run at reduced temperatures and pressures with low thermal energy requirement. Second, real-time products separation can be easily achieved with an electrochemical membrane reactor that keeps products generated at the cathode and anode separated. Moreover, the Faradaic efficiency (FE) of most electrochemical processes is close to unity, such as solid oxide fuel cell), resulting in high energy efficiency.
Technically, NG electrochemistry can be divided into two categories: room temperature electrochemistry (RTEC) and elevated temperature electrochemistry (ETEC). Typical cases of RTEC in chemical production are room temperature C2H4 production from CO2 reduction in aqueous solutions (Ren et al. 2015; DeWulf, Tuo, and Bard 1989; Hori et al. 1986) and proton exchange membrane fuel cell (PEMFC) with carbon-supported platinum catalyst.(Li et al. 2005) Since room temperature electrochemistry uses corrosive electrolyzer solution and precious metal as catalyst, the environmental issue and processing cost make it lack economic attractiveness. This topic is not covered in this section.
The solid oxide electrochemical cell (SOC), on the other hand, is suitable to operate at elevated temperatures to incorporate less expensive catalysts and is becoming an alternative for more efficient NG conversion (Wang et al. 2007b; Shi et al. 2008; Guo et al. 1997). Its advantages include: (i) the feedstock conversion rate is no longer subject to the thermodynamic equilibrium if one or more product species can be real-time separated (Champagnie et al. 1992); (ii) the potential to customize the thermodynamics and kinetics of the desired reaction with electrochemical assistance; and (iii) the much higher energy efficiency compared to the conventional high-temperature thermal cracking. We here narrow the discussion of NG electrochemical conversion to direct conversion of light alkanes (CH4, C2H6, and C3H8) based on the SOC technology, which is an emerging technology and attracts great technoeconomic interest from petrochemical industry.
1.2 Electrochemical Direct Conversion of Methane
Methane is a very stable, symmetrical molecule. The difference between indirect and direct methane conversion is the intermediate step of producing syngas (mixture of CO and H2) mainly from steam reforming or dry reforming. The direct conversion of natural gas into higher hydrocarbons has obvious advantage over the indirect way, as it simplifies the production procedure without producing syngas (Lunsford 2000). There are several possible routes for the direct methane conversion process, as shown in Figure 1.1. Methane pyrolysis is an equilibrium and thermal decarbonization reaction in the absence of oxygen to produce hydrogen and carbon black, which begins to produce carbon and hydrogen around 300°C and goes to completion around 1000°C. It is not a new process and has been employed for decades. However, there is no electrochemical method ever reported in this topic, making electrochemical methane decomposition an interesting area to explore for the continuous production of hydrogen and carbon with drastically reduced CO2 emissions. Electrochemical oxidation of methane into CO2 and water is typically achieved in methane fed fuel cells to produce electricity. There are several good review papers (Gür 2016; Choudhury, Chandra, and Arora 2013) on this topic and will not be discussed here.
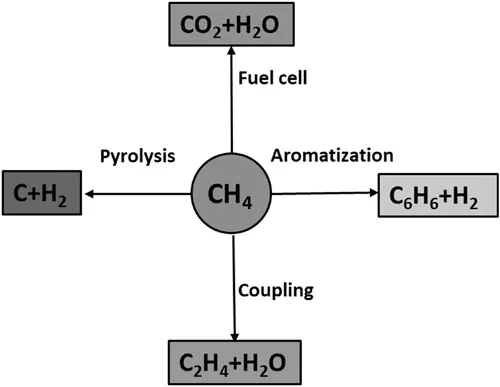
1.2.1 Electrochemical Methane Coupling to Produce Ethylene
The electrochemical methane coupling (EMC) process involves the reaction of CH4 and O2 over a catalyst at high temperatures to form C2H6 and/or C2H4. Conventionally, discussions about methane coupling involved co-feeding of reactants oxygen, steam, or carbon dioxide along with methane. In this section and hereafter, we only focus on oxygen as the only oxidative species to react with methane. To avoid confusion, the term “direct” methane conversion here implies that no reactants other than the methane is fed as feedstock.
There are two primary routes for the EMC process, depending on the existence of oxygen containing species in the feedstock: electrochemical oxidative methane coupling (EOMC) and electrochemical nonoxidative methane coupling (ENMC) processes. Figure 1.2 shows the operating principal schematic for both categories.
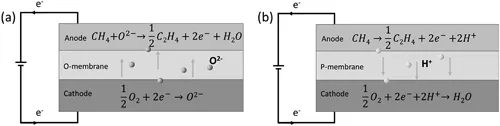
1.2.1.1 Electrochemical Oxidative Methane Coupling (EOMC)
In EOMC process, ...
Índice
- Cover
- Half-Title
- Title
- Copyright
- Contents
- Preface
- Editors
- List of Contributors
- Chapter 1 Electrochemical Conversion of Natural Gas to Value Added Chemicals
- Chapter 2 Microwaves in Nonoxidative Conversion of Natural Gas to Value-Added Products
- Chapter 3 Nonthermal Plasma Conversion of Natural Gas to Oxygenates
- Chapter 4 Natural Gas Conversion to Olefins via Chemical Looping
- Chapter 5 Oxidative Coupling of Methane
- Chapter 6 Direct Natural Gas Conversion to Oxygenates
- Chapter 7 Hydrogen and Solid Carbon Products from Natural Gas: A Review of Process Requirements, Current Technologies, Market Analysis, and Preliminary Techno Economic Assessment
- Chapter 8 Methane Conversion on Single-Atom Catalysts
- Chapter 9 Active Sites in Mo/HZSM-5 Catalysts for Nonoxidative Methane Dehydroaromatization
- Chapter 10 Natural Gas Dehydroaromatization
- Chapter 11 Multifunctional Reactors for Direct Nonoxidative Methane Conversion
- Chapter 12 Homogeneous Methane Functionalization
- Chapter 13 3D Printed Immobilized Biocatalysts for Conversion of Methane
- Chapter 14 Biological Conversion of Natural Gas
- Chapter 15 System Integration Approaches in Natural Gas Conversion
- Chapter 16 Techno-Economic Analysis of Microwave-Assisted Conversion Processes: Application to a Direct Natural Gas-to-Aromatics Process
- Index
Estilos de citas para Direct Natural Gas Conversion to Value-Added Chemicals
APA 6 Citation
Hu, J., & Shekhawat, D. (2020). Direct Natural Gas Conversion to Value-Added Chemicals (1st ed.). CRC Press. Retrieved from https://www.perlego.com/book/1693499/direct-natural-gas-conversion-to-valueadded-chemicals-pdf (Original work published 2020)
Chicago Citation
Hu, Jianli, and Dushyant Shekhawat. (2020) 2020. Direct Natural Gas Conversion to Value-Added Chemicals. 1st ed. CRC Press. https://www.perlego.com/book/1693499/direct-natural-gas-conversion-to-valueadded-chemicals-pdf.
Harvard Citation
Hu, J. and Shekhawat, D. (2020) Direct Natural Gas Conversion to Value-Added Chemicals. 1st edn. CRC Press. Available at: https://www.perlego.com/book/1693499/direct-natural-gas-conversion-to-valueadded-chemicals-pdf (Accessed: 14 October 2022).
MLA 7 Citation
Hu, Jianli, and Dushyant Shekhawat. Direct Natural Gas Conversion to Value-Added Chemicals. 1st ed. CRC Press, 2020. Web. 14 Oct. 2022.