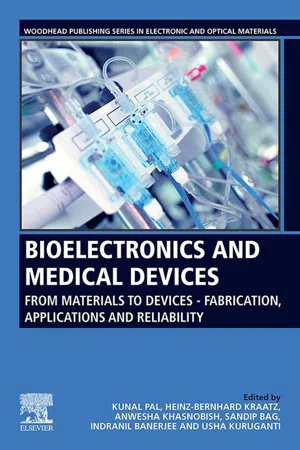
Bioelectronics and Medical Devices
From Materials to Devices - Fabrication, Applications and Reliability
Kunal Pal,Heinz-Bernhard Kraatz,Anwesha Khasnobish,Sandip Bag,Indranil Banerjee,Usha Kuruganti
- 1,006 páginas
- English
- ePUB (apto para móviles)
- Disponible en iOS y Android
Bioelectronics and Medical Devices
From Materials to Devices - Fabrication, Applications and Reliability
Kunal Pal,Heinz-Bernhard Kraatz,Anwesha Khasnobish,Sandip Bag,Indranil Banerjee,Usha Kuruganti
Información del libro
Bioelectronics and Medical Devices: From Materials to Devices-Fabrication, Applications and Reliability reviews the latest research on electronic devices used in the healthcare sector, from materials, to applications, including biosensors, rehabilitation devices, drug delivery devices, and devices based on wireless technology. This information is presented from the unique interdisciplinary perspective of the editors and contributors, all with materials science, biomedical engineering, physics, and chemistry backgrounds. Each applicable chapter includes a discussion of these devices, from materials and fabrication, to reliability and technology applications. Case studies, future research directions and recommendations for additional readings are also included.
The book addresses hot topics, such as the latest, state-of the-art biosensing devices that have the ability for early detection of life-threatening diseases, such as tuberculosis, HIV and cancer. It covers rehabilitation devices and advancements, such as the devices that could be utilized by advanced-stage ALS patients to improve their interactions with the environment. In addition, electronic controlled delivery systems are reviewed, including those that are based on artificial intelligences.
- Presents the latest topics, including MEMS-based fabrication of biomedical sensors, Internet of Things, certification of medical and drug delivery devices, and electrical safety considerations
- Presents the interdisciplinary perspective of materials scientists, biomedical engineers, physicists and chemists on biomedical electronic devices
- Features systematic coverage in each chapter, including recent advancements in the field, case studies, future research directions, and recommendations for additional readings
Preguntas frecuentes
Información
Light-fidelity based biosignal transmission
Abstract
Keywords
Introduction
Literature review
Índice
- Cover image
- Title page
- Table of Contents
- Copyright
- List of contributors
- 1. Light-fidelity based biosignal transmission
- 2. Development of a low-cost color sensor for biomedical applications
- 3. Development of a voice-controlled home automation system for the differently-abled
- 4. Lab-on-a-chip sensing devices for biomedical applications
- 5. Impedance-based biosensors
- 6. Acoustophoresis-based biomedical device applications
- 7. Electroencephalography and near-infrared spectroscopy-based hybrid biomarker for brain imaging
- 8. Micro-electro-mechanical system–based drug delivery devices
- 9. Enzyme-based biosensors
- 10. Ultrasound-based drug delivery systems
- 11. Electroencephalogram-controlled assistive devices
- 12. Electromyogram-controlled assistive devices
- 13. Electrical safety
- 14. Biomedical metrology
- 15. Bone-implantable devices for drug delivery applications
- 16. Iontophoretic drug delivery systems
- 17. Microneedle platform for biomedical applications
- 18. Trends in point-of-care microscopy
- 19. Development of spectroscopy-based medical devices for disease diagnosis in low resource point-of-care setting
- 20. Dielectrophoresis-based devices for cell patterning
- 21. Multichannel surface electromyography
- 22. Sensors for monitoring workplace health
- 23. Advances in enzyme-based electrochemical sensors: current trends, benefits, and constraints
- 24. Electrocardiogram signal processing-based diagnostics: applications of wavelet transform
- 25. Sensor fusion and control techniques for biorehabilitation
- 26. Biofunctional interfaces for cell culture in microfluidic devices
- 27. Microsystems technology for high-throughput single-cell sorting
- 28. Microfluidic devices for DNA amplification
- 29. Optimizing glucose sensing for diabetes monitoring
- 30. Brain–computer interface–functional electrical stimulation: from control to neurofeedback in rehabilitation
- 31. Motor imagery classification enhancement with concurrent implementation of spatial filtration and modified stockwell transform
- 32. A hybrid wireless electroencephalography network based on the IEEE 802.11 and IEEE 802.15.4 standards
- 33. Deep learning in medical and surgical instruments
- 34. Electroencephalogram-based brain–computer interface systems for controlling rehabilitative devices
- 35. A system for automatic cardiac arrhythmia recognition using electrocardiogram signal
- 36. Designing of a biopotential amplifier for the acquisition and processing of subvocal electromyography signals
- Index