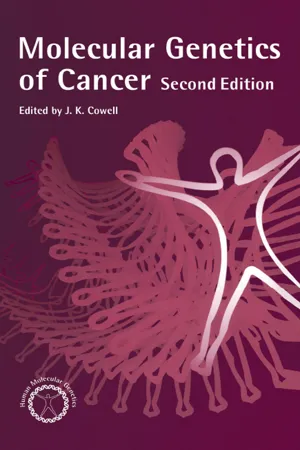
eBook - ePub
Molecular Genetics of Cancer
John Cowell
This is a test
- 536 pagine
- English
- ePUB (disponibile sull'app)
- Disponibile su iOS e Android
eBook - ePub
Molecular Genetics of Cancer
John Cowell
Dettagli del libro
Anteprima del libro
Indice dei contenuti
Citazioni
Informazioni sul libro
Molecular Genetics of Cancer, Second Edition provides an authoritative and up to date review of the key genes known to be critical in the development or progression of cancer. Throughout the book, scientific advances and their clinical relevance are covered in detail, particularly in the light of findings concerning the inheritance of genes predisposing to tumorigenesis. The book is therefore a valuable source of reference for clinicians and genetic counsellors as well as researchers.
Domande frequenti
Come faccio ad annullare l'abbonamento?
È semplicissimo: basta accedere alla sezione Account nelle Impostazioni e cliccare su "Annulla abbonamento". Dopo la cancellazione, l'abbonamento rimarrà attivo per il periodo rimanente già pagato. Per maggiori informazioni, clicca qui
È possibile scaricare libri? Se sì, come?
Al momento è possibile scaricare tramite l'app tutti i nostri libri ePub mobile-friendly. Anche la maggior parte dei nostri PDF è scaricabile e stiamo lavorando per rendere disponibile quanto prima il download di tutti gli altri file. Per maggiori informazioni, clicca qui
Che differenza c'è tra i piani?
Entrambi i piani ti danno accesso illimitato alla libreria e a tutte le funzionalità di Perlego. Le uniche differenze sono il prezzo e il periodo di abbonamento: con il piano annuale risparmierai circa il 30% rispetto a 12 rate con quello mensile.
Cos'è Perlego?
Perlego è un servizio di abbonamento a testi accademici, che ti permette di accedere a un'intera libreria online a un prezzo inferiore rispetto a quello che pagheresti per acquistare un singolo libro al mese. Con oltre 1 milione di testi suddivisi in più di 1.000 categorie, troverai sicuramente ciò che fa per te! Per maggiori informazioni, clicca qui.
Perlego supporta la sintesi vocale?
Cerca l'icona Sintesi vocale nel prossimo libro che leggerai per verificare se è possibile riprodurre l'audio. Questo strumento permette di leggere il testo a voce alta, evidenziandolo man mano che la lettura procede. Puoi aumentare o diminuire la velocità della sintesi vocale, oppure sospendere la riproduzione. Per maggiori informazioni, clicca qui.
Molecular Genetics of Cancer è disponibile online in formato PDF/ePub?
Sì, puoi accedere a Molecular Genetics of Cancer di John Cowell in formato PDF e/o ePub, così come ad altri libri molto apprezzati nelle sezioni relative a Medicine e Oncology. Scopri oltre 1 milione di libri disponibili nel nostro catalogo.
Informazioni
1
Basic principles in cancer genetics
1. Introduction
It is now well established that the malignant phenotype is genetically determined and that interactions between the environment and the genetic makeup of a cell can influence the course of specific tumors. In certain cases, mutations in specific genes confer a predisposition to cancer, again pointing to the importance of highly specific genetic changes in the development of particular tumors. As our understanding of the series of events which take place during the transformation of a normal cell into a cancer cell improves, it is becoming clear that cancer cells employ a complex repertoire of tricks, both to escape normal cellular growth control as well as overcome the powerful forces of terminal differentiation. The study of the genetic events which underlie this mechanism has been a priority of cancer molecular geneticists for many years, where the expectation is that, through a better understanding of the molecular genetic events that contribute to this commonly fatal collection of diseases, insights into novel therapies may emerge which, if not provide a cure, will hopefully retard the rampant growth of malignant cells that affects 1 in 4 adults and 1 in 800 children during their lifetimes.
Cancer genetics research, like the evolution of species, has progressed through major leaps, followed by consolidation in our understanding of the fundamental processes associated with these diseases. Although some of these major leaps resulted from provocative hypotheses, such as the two-hit theory (Knudson, 1971), more usually they coincided with individual technological advances such as chromosome banding (Caspersson et al., 1968; Seabright, 1971) or the polymerase chain reaction (Mullis and Faloona, 1987), which provided the means to test both new and old hypotheses. An accumulation of data then followed using these technologies in a rigorous, worldwide effort which, in turn, generated new hypotheses. The purpose of this opening chapter is to review some of the critical landmark developments in the field of cancer genetics which have allowed such amazing progress in our understanding of cancer development in such a relatively short time. By presenting the background and principles behind these developments, it is anticipated that it will then be possible to put the events leading to the isolation and characterization of human cancer genes in perspective.
When cancer cells divide they produce two cancer cells, clearly demonstrating that the malignant phenotype is genetically determined at the cellular level. The concept that cancer cells arise as a result of genomic instability is attributed to Theodore Boveri in 1914. Long before it was possible to clearly visualize chromosomes and almost half a decade before the structure of DNA was resolved, Boveri noted in his pathology practice that tumor cell nuclei showed mis-division and suggested that this chromosome instability was responsible for the development of cancer cells. He further suggested that random genetic events in a single cell caused chromosome abnormalities which conferred a permanent growth advantage and gave rise to clonal expansion with millions of similarly altered cells forming a malignancy. This hypothesis has proven to be remarkably accurate, and it has since been shown, that cumulative genetic changes give rise to tumor cells and that these changes may either be found in many cancer cells types or may be specific to a particular cell type.
2. Cytogenetics of cancer
One of the earliest developments in cancer research was the ability to be able to adequately separate and view the chromosomes from individual cells. For mammalian cells, analyzing chromosomes from ‘squash’ preparations was unsatisfying since the chromosomes were difficult to observe and count because of the individual overlaps. This problem was overcome with the development of hypotonic pre-treatments to swell the cells (Hsu, 1952) and chemical agents such as colchicine to disaggregate the spindle microtubules. This combined procedure made the cells fragile and so when dropped onto the microscope slide the cell burst releasing the chromosomes (Tjio and Levan, 1956). Immediately it was possible to count the chromosomes and arrange them not only into pairs of the same size but also to subgroup these chromosomes based on their centromeric position; e.g. telocentric (centromeres at the end), or metacentric (centromeres in the middle). This simple advance gave rise to countless studies of chromosomes in cancer cells describing numerical changes such as trisomies or monosomies for individual chromosomes (some of which are still used in diagnostics today), as well as large changes in chromosome numbers defined as pseudodiploidy, aneuploidy, hyperdiploidy and tetraploidy, depending on the extent of chromosome changes (Sandberg, 1990). Aneuploidy and hyperdiploidy, assessed either by chromosome number or DNA content measurement, is still used as a marker for tumor progression and in some cases may still be an adequate prognostic indicator (see Chapter 15). Large deletions within chromosomes could also be identified, although often it was not possible to establish unequivocally which chromosome was involved. An early, and very important, example of this was the report by Lele et al. (1963) of heterozygous constitutional chromosome deletions in retinoblastoma patients who showed a range of congential abnormalities including mental retardation. This chromosome change was in the so-called ‘D’ group of chromosomes, representing #13, #14 and #15. These studies laid the foundation for the subsequent search for, and cloning of, the retinoblastoma predisposition gene (Friend et al., 1986). A second classic example was the description of a recurrent, tiny marker – the so-called Philadelphia chromosome – in chronic myelogenous leukemia (Nowell and Hungerford, 1960) which turned out to be the consequence of a 9;22 translocation (Rowley, 1973). This observation was the prelude to the demonstration that translocations, in this case in leukemias, led to the generation of chimeric genes with novel functions. The major problem, however, at this point was that consistent chromosome changes in tumors could not be defined because of the inability to distinguish between the individual human chromosomes. This issue was resolved with the advent of chromosome banding techniques (Caspersson et al., 1968; Seabright, 1971). Using intercalating fluorescent dyes or proteases which selectively stripped proteins from fixed chromosomes, a characteristic linear distribution of light and dark bands along the length of the chromosomes could be generated. To the expert it was now possible to identify each of the human chromosomes based on their banding pattern and unequivocally identify each of the 23 different ones (Figure 1). The banding patterns also allowed subchromosomal changes such as deletions, translocations and inversion to be readily identified. Thus, began the ever increasing effort of characterizing cytogenetic changes in all types of tumor cells which, in turn, formed the basis for much of the molecular cloning era that followed.
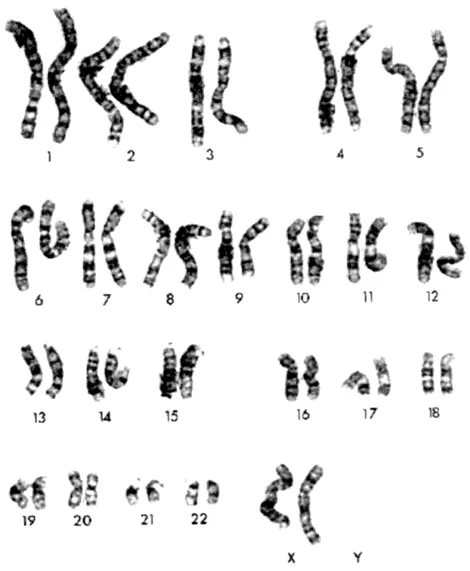
Figure 1. Example of a normal human female karyotype prepared from peripheral blood lymphocytes. Each of the chromosome pairs are arranged side by side based primarily on size and then on centromere position. The individual light-dark linear banding patterns are produced by mild trypsin treatment of acid-methanol fixed chromosome spreads followed by Giemsa staining. The G-band patterns for each of the chromosomes are quite distinct allowing unequivocal identification of each of the 23 different chromosomes (the Y chromosome is not shown).
Many cancer syndromes had already been defined clinically by the coincidence of a limited set of phenotypes, e.g the association of mental retardation with retinoblastoma (Lele et al., 1963), and aniridia with Wilms tumor (Riccardi et al., 1978). The ability to detect smaller and smaller changes, followed the development of new techniques to generate longer and longer chromosomes, pioneered in the laboratory of Jorge Yunis (Yunis, 1976). The ability to unequivocally identify each of the normal human chromosomes had also opened up the possibility of analyzing the constitutional chromosomes, e.g. in lymphocytes, from patients with cancer. It was subsequently shown that, in some of these cancer syndromes (Riccardi et al., 1978; Yunis and Ramsay, 1978), there were inherited chromosome abnormalities (Figure 2). Importantly these rearrangements not only pointed to the location of the genes responsible for the tumor predisposition but also provided a means of isolating them through positional cloning strategies (see below). This proved to be true in many cases and, in fact, the cloning of many cancer predisposition genes has been facilitated by these cytogenetic observations. As cytogenetic data accumulated, there became a need to catalog the various changes in order to identify consistencies between and within tumor types. This data provided a means to identify reliable markers which would not only aid in determining diagnosis and predict prognosis, but also to highlight regions within the genome that might contain genes important in the development of a particular malignancy. The task of doing this was adopted by Felix Mitelman and colleagues (Mitelman and Levan, 1981) who, after starting the catalog with an early report of approximately 1900 cytogenetic changes in tumor cells in 1981, now presides over an incredible volume describing over 100 000 changes and which is available for review online (Mitelman, 2000). These studies highlighted several phenomena in addition to the diversity of cytogenetic changes; firstly, that there were clearly consistencies in the regions of the chromosomes involved in abnormalities in specific tumor types. The consistency between tumors indicated that the rearrangements were important in tumorigenesis, especially where these represented the only cytogenetic change in the cells. Secondly, it was clear that the banding procedures could not always resolve the nature of every chromosome change and so valuable genetic information was un-interpretable. These unidentified chromosomes were banished to the end of the karyotype and simply described as ‘marker’ chromosomes to indicate their anonymous nature. Some of these marker chromosomes would turn out to be important and their characterization depended on yet another development in technology which was being developed in the late 1980s by groups such as the one headed by Dan Pinkel (Pinkel et al., 1986, 1988). These developments heralded the beginning of the era of ‘molecular cytogenetics’.
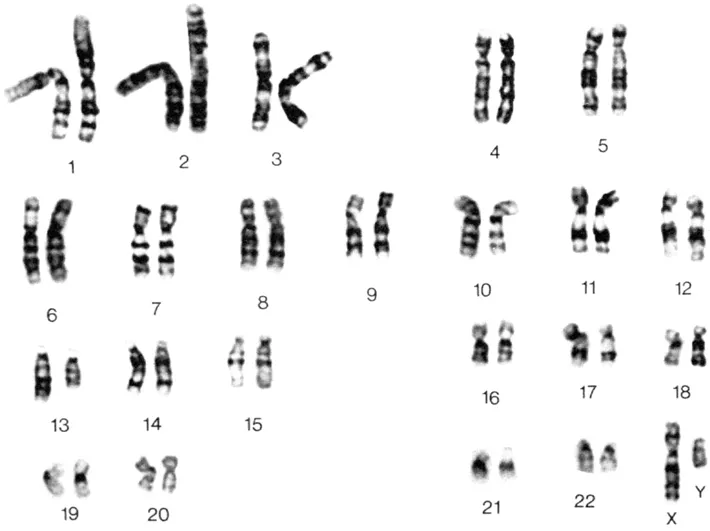
Figure 2. Giemsa-banded karyotype from peripheral blood lymphocytes from a patient with retinoblastoma and mental retardation. The only cytogenetic abnormality is a deletion within the long arm of one of the copies of chromosome 13.
3. The evolution of molecular cytogenetics
Although the hybridization of radioactively labeled DNA probes to chromosomes (in situ hybridization; ISH) was being applied to gene mapping, its use was mainly confined to repetitive regions of the chromosomes which could produce sufficient signal for detection. The ability to map unique sequences to particular regions of chromosomes was a more controversial issue. This was to change in the late 1980s as a result of several technological advances which proved to be essential for the molecular cytogenetics revolution: (1) the ability to label DNA probes with fluorochromes to high density; (2) the development of cameras which collected low levels of fluorescent light; (3) the construction of large insert genomic libraries (see below). The ability to suitably compete out repetitive sequences within these large probes (Landergent, 1987; Pinkel et al., 1988), together with the increased fluorescence signal obtained from large insert clones, provided the opportunity to localize markers directly on chromosomes in metaphase spreads. Thus, mapping by fluorescence in situ hybridization (FISH) became the primary way of localizing individual clones (Baldini et al., 1992). This approach also identified chimeric clones, since signal could be seen on two different chromosomes which proved to be an important piece of information for gene cloning experiments.
The technical advance which allowed this area to advance was the development and application of cooled, charge-coupled device (CCD) cameras which were able to capture very low light intensities emitted from the hybridizing clones. These cameras, therefore, which had been developed to stare out at distant galaxies where now being used to focus on some of the smallest structures within a single cell. In combination with software packages which digitally enhanced these images, this technology made visualization of probes relatively straightforward. Filter wheels capturing light from different emission spectra allowed several different probes to be used simultaneously and so their relative order could be determined. The advantage of this automated system was that light emissions were captured with minimal UV exposure and so the bleaching of the image, which is seen in regular fluorescence microscopy, was not a problem. This technique soon resulted in large databases of mapped probes, which made the identification of candidate genes associated with particular cancers much faster. The application of chromosome ‘painting’, which used complex probes (cDNA libraries, flow sorted chromosomes, Alu-PCR products) derived from single chromosomes or chromosome arms, to highlight specific chromosomes within a metaphase spread, overcame the need...
Indice dei contenuti
- Cover
- Half Title
- Title Page
- Copyright Page
- Table of Contents
- Contributors
- Abbreviations
- Preface
- 1. Basic principles in cancer genetics
- 2. Defining genetic changes associated with cutaneous malignant melanoma
- 3. The genetics of breast and ovarian cancer
- 4. Genetics of von Hippel-Lindau disease
- 5. Genetics of NF1 and NF2
- 6. Genetics of gastric cancer
- 7. Multiple endocrine neoplasia type 1
- 8. Multiple endocrine neoplasia type 2
- 9. TP53 in cancer origin and treatment
- 10. Colorectal cancer
- 11. Genetics of prostate cancer
- 12. Tuberous sclerosis complex
- 13. Retinoblastoma: patients, tumors, gene and protein contribute to the understanding of cancer
- 14. Genetics of Wilms tumor
- 15. Genetics of neuroblastoma
- 16. Genetics of brain tumors
- 17. Molecular genetics of lung cancer
- 18. Genetics of liver cancer
- 19. The genetics of bladder cancer
- 20. Genetics of rhabdomyosarcoma
- 21. Role of telomeres and telomerase in aging and cancer
- Index
Stili delle citazioni per Molecular Genetics of Cancer
APA 6 Citation
[author missing]. (2003). Molecular Genetics of Cancer (1st ed.). CRC Press. Retrieved from https://www.perlego.com/book/1711055/molecular-genetics-of-cancer-pdf (Original work published 2003)
Chicago Citation
[author missing]. (2003) 2003. Molecular Genetics of Cancer. 1st ed. CRC Press. https://www.perlego.com/book/1711055/molecular-genetics-of-cancer-pdf.
Harvard Citation
[author missing] (2003) Molecular Genetics of Cancer. 1st edn. CRC Press. Available at: https://www.perlego.com/book/1711055/molecular-genetics-of-cancer-pdf (Accessed: 14 October 2022).
MLA 7 Citation
[author missing]. Molecular Genetics of Cancer. 1st ed. CRC Press, 2003. Web. 14 Oct. 2022.