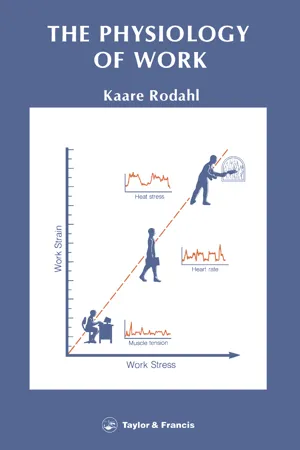
eBook - ePub
Physiology Of Work
Kaare Rodahl
This is a test
Share book
- 296 pages
- English
- ePUB (mobile friendly)
- Available on iOS & Android
eBook - ePub
Physiology Of Work
Kaare Rodahl
Book details
Book preview
Table of contents
Citations
About This Book
This text focuses on the applied physiology of work in modern industry. After covering the biological background to work physiology and its relationship to work psychology and occupational medicine it goes on to explore the problems encountered via case studies. Rodhal describes methods for assessment of individual work capacities and workloads, and evaluation of working environments. Further chapters highlight: mental and emotional stresses, including case studies from management and air-traffic controllers; industrial heat and cold stress, with studies of polar and sea-going workers; and problems encountered in polluted atmospheres.
Frequently asked questions
How do I cancel my subscription?
Can/how do I download books?
At the moment all of our mobile-responsive ePub books are available to download via the app. Most of our PDFs are also available to download and we're working on making the final remaining ones downloadable now. Learn more here.
What is the difference between the pricing plans?
Both plans give you full access to the library and all of Perlego’s features. The only differences are the price and subscription period: With the annual plan you’ll save around 30% compared to 12 months on the monthly plan.
What is Perlego?
We are an online textbook subscription service, where you can get access to an entire online library for less than the price of a single book per month. With over 1 million books across 1000+ topics, we’ve got you covered! Learn more here.
Do you support text-to-speech?
Look out for the read-aloud symbol on your next book to see if you can listen to it. The read-aloud tool reads text aloud for you, highlighting the text as it is being read. You can pause it, speed it up and slow it down. Learn more here.
Is Physiology Of Work an online PDF/ePUB?
Yes, you can access Physiology Of Work by Kaare Rodahl in PDF and/or ePUB format, as well as other popular books in Technologie et ingénierie & Ingénierie industrielle. We have over one million books available in our catalogue for you to explore.
Information
Chapter 1
The biological basis for human work
The human body is made for physical work. This was true in the case of the Stone Age hunter, and it is still the case in the present-day technocrat, for by all indications, the human body has not changed significantly during the last 100, 000 years. We are still born with a mass of muscles equivalent to more than half our body weight for the purpose of carrying our body around and for doing muscular work. These muscles are capable of transferring chemical energy in the food which we eat, into mechanical work, with a mechanical efficiency comparable to that of a modern automobile engine. These muscles are not only capable of developing great muscular strength and of carrying loads equivalent to that of our own body weight, they are also capable of generating a wide range of tensions necessary when moving different parts of our body, especially the extremities, and manipulating minute objects, as in the case of electronic technology.
We are, like all higher animals, basically designed for mobility, which is the basic requirement for the performance of any kind of physical work. Consequently, our locomotive apparatus, i.e. the neuromuscular-skeletal system, constitutes the majority of our total body mass.
The muscle, which is the basic instrument of mobility, is a very old tissue. The earliest fossil traces of animal life were burrows which began to appear in rocks almost 700 million years ago (Valentine, 1978). Evidently, these animals could dig in the sea bed, using muscle force. The energy-yielding processes in these muscle cells were the same as those occurring in the first unicellular organisms some 1,500 million years ago, and as those occurring in our muscle cells today (for references see Åstrand and Rodahl, 1986).
Of all the tissues in our body, the muscle is unique in that it can vary its metabolic rate to a greater extent than any other tissue, when changing from rest to work. Skeletal muscles may increase their oxidative processes some 50 times the resting level. While this level of exertion is typical of many types of athletic events, it is hardly ever encountered in ordinary physical work or labour, where the energy metabolism seldom exceeds 25 per cent of the maximal attainable level.
Another feature of the muscle cell is that it, similar to certain other cells, such as nerve cells, is not replaced if destroyed. Contrary to cells such as connective tissue cells, muscle cells do not regenerate. They can, however, increase in thickness by training, and thus develop greater strength.
The supply of blood, and thereby the supply of nutrients and oxygen, to the working muscle cell is determined by the amount of work the muscle is doing. When resting, the smooth muscle fibers constricting the blood capillaries supplying the skeletal muscle tissue, are contracted, keeping the capillary lumen nearly closed, so that the muscle cells only receive a minimum of blood, sufficient to stay alive. When working, however, the active muscle cells produce metabolites which relax the smooth capillary muscles, and allow more blood to pass into the muscle tissue; and more so the heavier the muscle is working. This is an example of one of nature’s decentralized, democratic regulatory mechanisms based on the principle that the muscle cells which are most active receive the greatest share of the available blood supply. Thus, in the case of the muscle, it pays to work: the more they do, the more they get.
Some 10–20 million years ago our forerunners, the hominids, developed as a separate branch from the family tree of primates. When they abandoned their life in the trees and started to forage and hunt on the ground, they initiated a bipedal adaptation to terrestrial life (Valentine, 1978). Their pelvis permitted an upright posture with a bipedal gait, which freed their arms and hands for carrying their necessities with them as they walked. Tool-making was probably established more than three million years ago, before any marked brain expansion had occurred (Lewin, 1981). By the time of the Neanderthal man appearing more than 100,000 years ago, the same postural abilities, manual dexterity, and range and character of movement typical for modern man, had been developed. Up to that time the increase in brain size seems to be correlated with the increasing complexity of stone tools (Washburn, 1978).
Just as the proportions of the human hand, with its opposable thumb, is ideally suited for manual work and the use of tools, so does the anatomy of the human brain reflect a successful adaptation for manual and intellectual skills. The superiority of the human brain is clearly evidenced by the proportion of the total energy production devoted to the central nervous system. While adults of most vertebrate species devote less than 8 per cent of their basal metabolism to the central nervous system, our central nervous system consumes 20 per cent of our basal metabolism (Mink et al., 1981). The superior human brain capacity not only makes us ideally suited for intellectual work, but also highly suited for skilled manual and physical performance. Consequently, an exceptionally large area of our motor cortex is devoted to the motor control of our hands and fingers. It is a remarkable fact that from all indications this is not a recent evolutionary feature. Most likely, a human being living more than 50,000 years ago had the same potential for physical and intellectual performance, including constructing and operating a computer, as anyone of us living today.
It thus appears that ancient man, as well as modern man, was basically made to employ his body physically in his struggle for survival. Man, like all higher animals, reacts to almost any kind of a threat by mobilizing his resources for a physical effort, i.e. by the so-called stress reaction. The sight or awareness of any kind of threat automatically evokes nervous and hormonal reactions, mobilizing the bodily resources for a physical response to the threat (Cannon, 1929). The pulmonary ventilation increases as does the heart rate and cardiac output, increasing the oxygen uptake and the oxygen delivery to the muscles, in readiness for work. Much of the circulating blood volume is shifted from the guts and skin to the muscles. Due to the effect of some of the stress hormones liberated as a consequence of the stress mechanism, stored energy is released in the form of glucose and free fatty acids, now being made available as substrates for the working muscles. At the same time the excreted stress hormone Cortisol exerts a profound effect on a number of cellular functions, including cellular membrane permeability and the breakdown of proteins to carbohydrates to be used as metabolic fuel for the working muscles (for references, see Malm, 1969; Francis, 1979; Åstrand and Rodahl, 1986).
As already pointed out by Cannon (Herd, 1972), the purpose of all these changes, brought about by the stress reaction serving as a trigger mechanism, is primarily to prepare the organism for a physical effort to cope with the threatening situation—as was the case when a Stone Age hunter suddenly encountered a threatening bear in the wilderness. As soon as the threatened individual engaged himself in the physical activity which the situation called for, be it a fight with the bear or a run for safety, the inherent physiological regulatory mechanisms of the stressed body took over, adjusting the regulatory organ systems to meet the need of the body in terms of physical performance, i.e. pulmonary ventilation, cardiac output, blood volume distribution, fuel mobilization, temperature regulation, etc. Similarly, as soon as the effort was over, the same regulatory mechanisms brought the excited organism back to a state of rest and recuperation (Åstrand and Rodahl, 1986).
Our problem is that any stressful situation in our civilized existence may trigger the same stress reaction as in our primitive ancestor confronted with a wild beast, but without being able to achieve a physical outlet for our stress response. This, in the long run, may cause ill effects, including gastro-intestinal upset and elevated blood pressure (see Chapter 6).
If it may be assumed that the remaining primitive groups of people— such as the Bushmen in Africa, the Aborigines in Australia and the Eskimos in the Arctic—reflect the behaviour of our original ancestors, a great deal can, be learned about our functional biology from watching them and the way they engage their endowed body potential in their struggle for survival. One feature which they all seem to have in common is that they move about at a fairly moderate pace, when they hunt or gather food. With great endurance they may stand or sit patiently for hours waiting for the game to appear, but seldom run, at any rate not for very long distances. This is in direct contrast to our own emphasis on the practice of athletic activities such as prolonged jogging or even marathon running. It is true that the Eskimos, and especially the Eskimo children and youngsters, may engage in many kinds of competitive sports, but in most cases they involve brief spurts of dynamic activity, interspaced with periods of rest and relaxation. Perhaps the most vigorous and protracted athletic performance of the Eskimo is their drum dance, a highly-dynamic and varied physical activity.
Basically, most of our inherent physiological functions involve rhythmic, dynamic activity, interspaced with inactivity or relaxation, i.e. rhythmic contraction and relaxation of the respiratory muscles as we breath, the cardiac muscle pumping the blood, the skeletal muscle as we walk, the smooth muscles of the gut causing peristaltic movement of its content, and the repeated electrical discharges in the nervous system causing the initiation and propagation of the nervous impulses.
It is thus evident that our body machinery is ideally suited for dynamic work in which exertion and relaxation interchange more or less rhythmically, with a varied use of the different parts of the body, both physical and mental. In the final analysis this may even apply to the interplay between physical and mental engagement. While this type of varied body use was commonplace among our primitive ancestors, it is certainly not so in the case of more sedentary factory workers, such as those engaged in electronic assembly work. The food forager was free to move about, using his eyes to view the horizon or to see where he was going, moving his head and his extremities in a varied way, changing the load on his muscles and constantly alternating the use of his body. In this manner he was able to rest parts of his body while engaging other parts as he proceeded on his way. In contrast to this, our modern electronic assembly line operator, often a woman, usually has to focus her attention on the minute microchips or other parts in front of her. In order to keep the tiny part in sight, she has to keep her eye muscles constantly tensed, and her head fixed, necessitating a constant tensing of the muscles of her neck. In order to move the delicate instruments manipulating the minute parts that she is working on, she has to keep the muscles of her shoulder, arms and hands fixed and tensed all the time, perhaps for hours, day after day, month after month. This sooner or later may lead to pain which in some cases may be the cause of disabling complaints and prolonged sick leave, and varying degrees of disability.
A study of an electronic assembly plant in Norway (Westgaard et al., 1984), revealed that 60 per cent of the healthy young girls starting to work in the plant developed muscular-skeletal complaints causing sick absenteeism for three weeks or more at the end of two and a half years. Even when the work place and the work procedures were improved to meet all ergonomie requirements, the symptoms still developed, the only difference being that they occurred a little later.
The conclusion which may be drawn from this brief review of the biological basis for human performance is that we are ideally suited for varied dynamic work, and that many of the problems of the present-day worker are the consequences of too much static work and stress, with which we are unable to cope.
Chapter 2
Work physiology
Originally, the science of physiology was largely concerned with the function of the healthy organism at rest. Only in recent decades has the student of physiology and medicine been concerned, to any extent, with the function of the human body under the stress of work.
Yet, there were exceptions. Probably one of the very first to emphasize the importance of the study of work, as a means to attaining safe and proper working conditions, was the Polish educator Wojciech Jastrzebowski, who died in 1882 (Polish Ergonomics Society, 1979). During the early part of this century a few of the physiologists in Europe, especially in Germany and England, were actively engaged in the study of several fundamental aspects of the physiology of work and exercise. The first Institute of Work Physiology was founded by Max Rubner in Berlin in 1913, ‘Kaiser-Wilhelm-Instiut für Arbeits-physiologie’ (Rutenfranz et al., 1981), later (1929) known as the Max-Planck-Institute of Work Physiology in Dortmund, under the leadership of Gunther Lehmann, the author of one of the first major textbooks of Applied Work Physiology (Lehmann, 1953). Significant contributions to this field were also made by A.Krogh and his co-workers in Copenhagen, eventually extending to the other Scandinavian countries. In Great Britain, A.V.Hill made a series of fundamental contributions to the basic understanding of the functioning of the human living machinery’ from 1925 to the beginning of World War II (Jokl, 1980). In the USA a group headed by D.B.Dill formed the Harvard Fatigue Laboratory which made significant contributions to the field of work physiology until it was dissolved during the Second World War.
During the war, applied human factors scientists became involved in what was called human engineering. They were trying to adjust physical facilities, such as the aircraft cockpit to fit the human body which was to occupy it, and arranging the instrument panel in a manner most suitable for the operator in terms of convenience and efficiency. Many of them were psychologists, but the working team might also include physiologists, anthropologists and engineers.
This type of activity continued after the war both in the USA and Europe under the name of Human Factors Research, mostly in military and aerospace laboratories. The problem areas included simulator operations, cockpit design, communication systems, stress response, etc. In the early 1950s this became known as ergonomics, a term which by some workers in the field is taken to mean more or less anything that has to do with work, including the entire subject of work physiology, work psychology, as well as human factors and human engineering.
During the Second World War some of the extreme environments facing the fighting soldier (such as the heat of the desert), or the sailor (such as the operation of manned submarines) captured the interest of many capable physiologists (see Adolph, Physiology of Man in the Desert, 1947, as an example). This type of occupational and environmental physiology under rather extreme conditions (Arctic warfare, altitude and underwater operations) attracted considerable support and recruitment also during the first few decades after the war. This was especially the case during the Cold War prior to the development of intercontinental missiles when the shortest distance by air between the two opposing superpowers was across the Arctic Ocean. At that time, cold weather operations and Arctic survival were matters of prime importance. This brought out the need for a better insight into the factors affecting human cold-weather operations. Consequently, funds in support of these aspects of work and environmental physiology became abundantly available. As a result of this, groups of very capable work physiologists were formed in a number of well-equipped laboratories in several countries around the world. Eventually, these groups contributed significantly to the understanding of such fundamental problems as temperature regulation, the physiological reaction to cold exposure, etc. When the intercontinental ballistic missiles more or less replaced the need for large numbers of Arctic ground and air forces in constant combat readiness, the need for research on temperature regulation and related fields was no longer obvious, and much of the support for this aspect of work physiology was discontinued. As a consequence, the research effort in these areas has remained at a fairly low level world wide, and the progress made since the active period during the 1950s is comparatively modest.
In its early stages of development, work physiology included both the physiology of physical work or labour, and the physiology of athletic performance. It was all considered work in terms of the basic definition of the term: application of force through a distance. In those days when heavy physical work, taxing a sizeable part of the individual’s maximal work capacity, was still commonplace, this concept appeared logical. Consequently, a great deal of emphasis was placed on the energy cost of physical work, and the corresponding requirement for dietary energy intake, rest pauses, endurance, movement (kinesiology), the assessment of maximal physical work capacity, and the fraction of this capacity which was being taxed in the performance of the work operation in question (for references see Åstrand and Rodahl, 1986). Since the same organ functions were involved, both in physical labour and athletic exercise, it was natural to consider both disciplines as one.
This may no longer be equally true, since very heavy physical work no longer is generally encountered in manual labour. In fact, work loads in excess of 30–40 per cent of the individual’s maximal work capacity are very rare. Furthermore, the metabolic events, and the organic stress at the cellular level, are entirely different in the two situations: ordinary manual labour, and competitive athletic exercise. This was already pointed out by Courtice and Douglas in 1936, when they wrote:
‘In everyday life the muscular work which we are called upon to perform is done at a far lower rate than that which is done by the athlete who is pushing himself almost to the limit of his power. It by no means follows that the facts observed during severe exercise, and the deductions made from these, can be applied without alteration when we wish to explain the effects of exercise of far less severity.’
It is therefore perhaps not surprising that those studying the physiology of athletes in recent years have insisted on distinguishing between the physiology of work in terms of physical labour, and the physiology of exercise in terms of athletic performance. Of these two research areas, the physiology of exercise has attracted greater interest in terms of recruitment of research workers than has the physiology of work.
Whereas work physiology was originally, to a large extent, concerned with heavy physical work and labour, this is, as already pointed out, no longer the case. Much of the heavy physical labour is already eliminated and much of what is left can quite easily be taken over by mechanical aids.
In this process of abolishing heavy, dynamic physical work, we have gradually replaced it with prolonged static work, using relatively small muscle groups, often in a fixed position, at an intense working rate, involving considerable mental tension and requiring a high degree of accuracy, a stress situation the human organism is not ideally equipped to endure or to cope with.
During recent decades ergonomists have made admirable efforts to improve the working conditions in keeping with the limitations of the human capabilities. They have improved the arrangement of the actual work place in terms of working posture, reach, table heights, and have adjusted the tools to fit the worker who is using them. Yet the net result is an increasing number of workers suffering varying degrees of muscular-skeletal disorders from painful, transient muscle tension, to chronic disabling fibromyalgia. This is the result of exposing the working individual to a type of static, monotonous, prolonged, and repetitive work, at a high level of maintained concentration and neuromuscular tension, for which the human mind and body is not suited. Consequently, there is a limit to how much the ergonomists may achieve in terms of preventive measures. They may, in fact, in some cases, already have reached the limit, in spite of having developed an awareness on the part of the working individual as to how to sit and how to use the tools, etc., for the symptoms still appear in an ever-increasing number. Consequently, instead of preventing these ill effects, the ergonomist is now, to an increasing degree, involved in the treatment of the symptoms of a faulty use of the human body as a working machinery.
Now the major problem, and indeed the most costly one in t...