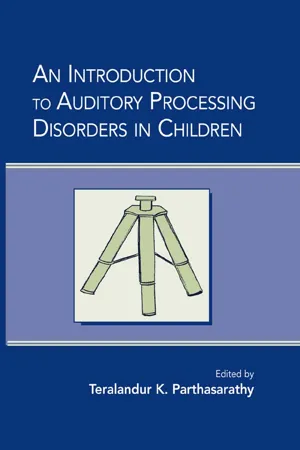
eBook - ePub
An Introduction to Auditory Processing Disorders in Children
Teralandur K. Parthasarathy, Teralandur K. Parthasarathy
This is a test
Share book
- 306 pages
- English
- ePUB (mobile friendly)
- Available on iOS & Android
eBook - ePub
An Introduction to Auditory Processing Disorders in Children
Teralandur K. Parthasarathy, Teralandur K. Parthasarathy
Book details
Book preview
Table of contents
Citations
About This Book
Auditory processing in children (APD) comprises an increasingly important clinical area within the broad field of communication disorders. This new textbook presents the major advances in the assessment and management of APD. The chapter authors, highly regarded clinicians and researchers from diverse professional groups, contribute an impressive breadth of knowledge to explain and demystify APD. This text will be useful to students of speech language pathology and audiology, as well as professionals in those fields.
Frequently asked questions
How do I cancel my subscription?
Can/how do I download books?
At the moment all of our mobile-responsive ePub books are available to download via the app. Most of our PDFs are also available to download and we're working on making the final remaining ones downloadable now. Learn more here.
What is the difference between the pricing plans?
Both plans give you full access to the library and all of Perlegoās features. The only differences are the price and subscription period: With the annual plan youāll save around 30% compared to 12 months on the monthly plan.
What is Perlego?
We are an online textbook subscription service, where you can get access to an entire online library for less than the price of a single book per month. With over 1 million books across 1000+ topics, weāve got you covered! Learn more here.
Do you support text-to-speech?
Look out for the read-aloud symbol on your next book to see if you can listen to it. The read-aloud tool reads text aloud for you, highlighting the text as it is being read. You can pause it, speed it up and slow it down. Learn more here.
Is An Introduction to Auditory Processing Disorders in Children an online PDF/ePUB?
Yes, you can access An Introduction to Auditory Processing Disorders in Children by Teralandur K. Parthasarathy, Teralandur K. Parthasarathy in PDF and/or ePUB format, as well as other popular books in Education & Inclusive Education. We have over one million books available in our catalogue for you to explore.
Information
1
Neuroanatomy and Neurophysiology of the Central Auditory Pathways
HEARING SENSITIVITY
Normal hearing is essential to the acquisition of oral language and effective verbal communication. Any impairment of the auditory system, either congenitally or adventitiously acquired, that affects the transmission and/or perception of sound is likely to have a profound effect on one's ability to hear and comprehend spoken language. The human ear is sensitive to acoustic events within the frequency range of 20 to 18,000 Hertz (Hz). However, the human ear is not equally sensitive to all frequencies within this range. The ear is most sensitive to sound frequencies between 500 and 4,000 Hzāthe frequency range most important for speech reception. In terms of intensity, the human auditory system is sensitive to a range of 0 to 140 dB Sound Pressure Level (SPL). Normal conversational speech falls within the range of approximately 50 to 77 dB SPL. Repeated and prolonged exposure to intensity levels above 85 dB SPL can cause permanent structural damage to the inner ear. Sound pressure levels above 140 dB SPL can cause pain sensation and instantaneous structural damage to the hearing mechanism.
OVERVIEW OF AUDITION
The process of audition (hearing) begins when sound waves enter the external auditory meatus and impinge on the tympanic membrane. The subsequent movement of the tympanic membrane serves to convert sound energy into mechanical energy. The motion (vibration) of the tympanic membrane causes the middle ear bonesāmalleus, incus, and stapesāto be set into motion. This mechanical energy is transmitted to the cochlear fluids of the inner ear via stapes movement in and out of the oval window of the inner ear, thus converting mechanical energy into hydraulic energy. The resulting movement of the fluids causes patterned membrane movement in the cochlea. Movement of the membranes in the cochlea stimulate the cilia of the hair cells, which in turn causes depolarization of the hair cells. Depolarization activates chemical channels, triggering the release of neurotransmitters, across the synapse between the hair cells and auditory nerve fibers. The neurotransmitter depolarizes the terminals of the auditory nerve fiber and a nerve action potential is generated. The action potential discharges are transmitted by the fibers of the auditory nerve to the cochlear nuclei in the brain stem, which project these nerve impulses to multiple synaptic points in the brain stem as well as the thalamus. The combined signals from both ears are analyzed in the brain stem by their intensity and frequency to localize sound. Auditory impulses finally travel to the primary auditory cortex located on the superior surface of the temporal lobe in the gyri of Heschl, which are involved with sound perception. The auditory impulses further travel to Wernicke's (associational language) area, where the auditory signals are analyzed and interpreted into language-specific meaningful messages and the comprehension of spoken language occurs.
This chapter provides a functional description of the neural circuitry and physiology of hearing from the inner ear to the primary auditory cortex. Each intersecting level of the brain stem is listed and functionally discussed in terms of its significance to the transmission of auditory information.
INNER EAR MECHANISM
The inner ear contains the sensory organs of balance and hearing. The sensory organ for balance is called the vestibular system and includes the utricle, saccule, and three semicircular canals. The sensory organ for hearing is called the acoustic or auditory system. The auditory portion of the inner ear is a snail-shaped structure called the cochlear. Both the vestibular and auditory systems are encased in the same bony capsule, contain the same fluid systems, and share the same cranial nerveāCranial nerve VIII (Fig. 1.1).
There are two labyrinths in the inner ear: the osseous (bony) labyrinth and the membranous labyrinth. The osseous labyrinth is a bony capsule covering a series of irregular cavities in the petrous portion of the temporal bone. Inside the osseous labyrinth is the membranous labyrinth, which includes the cochlear duct (scala media) and the vestibular apparatus of saccule, utricle, and three semicircular canals. The inner ear fluid perilymph protects the membranous labyrinth from the bony labyrinth. Endolymph is a closed fluid system within the membranous labyrinth of the cochlear and vestibular portions of the inner ear.
COCHLEAR ANATOMY
The cochlea resembles a snail-shaped structure winding two and a half times around a bony, central core called the modiolus. A cross-section of the cochlea is depicted in Fig. 1.2.
With a length of approximately 35 mm, the cochlea is comprised of three fluid-filled chambers or canals: scala vestibuli, scala media, and scala tympani. Scala vestibuli is the uppermost chamber and follows the inner contour of the cochlea. Scala tympani lies at the bottom and follows the outer contour of the cochlea. Scala vestibuli communicates with scala tympani through a small aperture at the apex of the cochlea called the helicotrema.
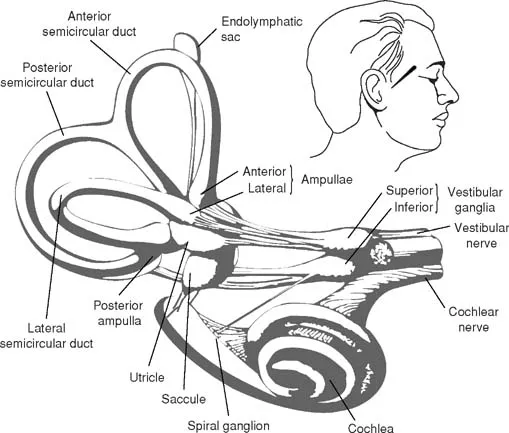
FIG. 1.1. Inner ear labyrinth composed of the semicircular ducts, vestibule, and cochlea along with vestibular and cochlear nerves. From Neuroscience for the Study of Communicative Disorders, 2nd. ed. by Subhash C. Bhatnagar, 2001. Copyright 2001 by Lippincott, Williams and Wilkins.
Reisner's membrane, or vestibular ligament, separates scala vestibuli from scala media. The basilar membrane separates scala media from scala tympani. The end organ of hearingāthe organ of Cortiāis supported by the basilar membrane. Both membranes extend from the osseous (bony) spiral lamina, a bony shell that extends from the modiolus, and attach to the spiral ligament located on the wall of the bony labyrinth.
Scala vestibuli and scala tympani are filled with perilymph, which is secreted by the periosteal lining of the scalae. Scala media is filled with endolymph, which is secreted by stria vascularis, a highly vascularized band of cells on the internal surface of the spiral ligament within scala media. Both of these fluids are marked by different concentrations of sodium and potassium ions. The higher sodium (Na+) relative to the lower potassium (K+) concentration of perilymph makes it similar to cerebrospinal fluid (CSF) or extracellular fluid. The ionic composition of endolymph, which has a higher concentration of K+ relative to Na+, is similar to the intracellular fluid (Haines, 2002).
The organ of Corti, which is located in the scala media on the basilar membrane, contains the sensory cells of hearingāthe hair cells. The hair cells are equipped with specialized stereocilia on their tips. There are two types of hair cells: inner and outer (Fig. 1.2). In humans, these sensory cells run in parallel rows from the base of the cochlea to the apex of the cochlea. Inner hair cells (IHC) run in a single row, outer hair cells (OHC) in rows of three or four. The arch of Corti separates the row of IHC from the rows of OHC. There are approximately 12,000 OHCs and 3,000 IHCs. Fifty to 70 stereocilia project from each IHC, forming a āVā pattern. Forty to 150 stereocilia project from each OHC, forming a āWā pattern. The cilia of the OHCs fit into indentations on the underside of the overlying gelatinous tectorial membrane. The cilia of the IHC do not directly insert into the tectorial membrane.
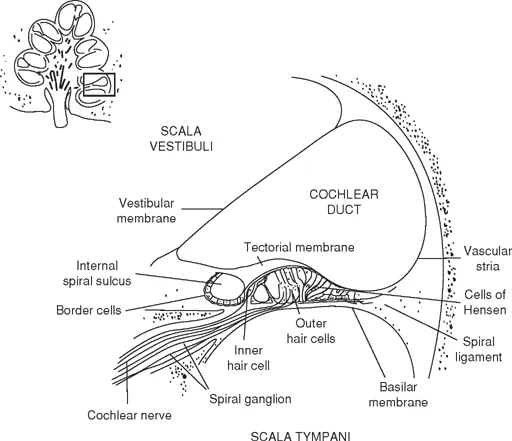
FIG. 1.2. A diagrammatic illustration of the cochlear duct on a radial section of the cochlea. From Neuroscience for the Study of Communicative Disorders, 2nd. ed. by Subhash C. Bhatnagar, 2001. Copyright 2001 by Lippincott, Williams and Wilkins.
IHC and OHC differ in terms of their innervations: IHC are predominantly innervated by ascending auditory nerve fibers, whereas the OHC are predominantly innervated by projections from the descending auditory pathways including the olivocochlear bundle (OCB).
COCHLEAR FUNCTION
The cochlea converts the mechanical energy received from the middle ear ossicles into hydraulic mechanical energy and initiates neural activity. As the stapes moves into the oval window, it displaces perilymph toward scala tympani through the helicotrema. Due to the incompressibility of fluids of the cochlea because of the surrounding bony labyrinth, the resulting fluid pressure is relieved by the outward movement of the round window in the scala tympani. As the stapes moves out of the oval window, perilymph is displaced toward the scala vestibuli with a corresponding inward movement of the round window. Because the basilar membrane (BM) is structurally flexible, it responds to this pressure by its own displacement. As the basilar membrane is displaced beginning at its base, the deformation moves toward the apex of the cochlea as a traveling wave. As the wave moves toward the apex of the cochlea, its velocity slows but the amplitude increases. Amplitude of basilar membrane displacement reaches a maximum at a specific point along the membrane before gradually attenuating. The point of maximum amplitude of basilar membrane displacement corresponds to the frequency of the stimulus. Thus, different sound frequencies produce different traveling wave patterns, forming peak amplitudes at different regions of the cochlea. Maximum amplitude of the basilar membrane traveling wave occurs near the base of the cochlea for high-frequency sounds. As the frequency of the stimulus decreases, the peak amplitude of the traveling wave moves toward the apex of the cochlea. A signal consisting of many frequencies will cause a traveling wave with multiple peaks along the basilar membrane.
The hair cells located at the point of the maximum basilar membrane movement are the most stimulated, suggesting that cochlear frequency selectivity is initially related to the mechanical properties of the basilar membrane. However, the frequency selectivity also may be related to structural and electrical properties of hair cells. This relationship between the maximum cochlear response and characteristic frequency is the basis of the place theory of hearing, and this tonotopic organization is preserved throughout the auditory system.
The deformation of the basilar membrane results in the mechanical displacement of the cilia of the hair cells by the overlying tectorial membrane. The mechanical displacement of the ciliaāor shearing actionāleads to the depolarization of the hair cells. The depolarization triggers the release of neurotransmitters from the synaptic vesicles at the base of the hair cells. The neurotransmitters have a depolarizing effect on the terminals of the auditory nerve fibers that results in the generation of action potentials which travel to the brain stem.
ELECTRIC TRANSDUCTION
The cilia of the hair cells are embedded in the endolymph, which has an electrical potential of +80 mV. This endolymphatic potential is supplied by stria vascularis. Stimulation of the cilia of the hair cells allows this potential to flow through the hair cell, initiating neural activity in the cochlea. Genetic or acquired structural pathology of stria vascularis is likely to alter the biochemical process for nerve impulses (Haines, 2002).
Specifically, the initiation of neural activity in the cochlea is a result of the ionic properties of the hair cells and the transmission of charged particles through the hair cell membranes. The presence of a ā70 mV intracellular environment and +80 mV endolymphatic potential in scala media results in a 150 mV gradient difference across the apical ends of the cilia. This difference serves to regulate the electrical response of hair cells to mechanical deformation.
Basilar membrane movement deforms the stereocilia against the tectorial membrane, and this results in a graded depolarization of the hair cells. Deformation of the stereocilia of hair cells increases potassium (K+) permeability and opens K+ sensitive pores in the tips of cilia. The inward K+ current enters the hair cells through the cilia and depolarizes the hair cells. This depolarization also opens the voltage-sensitive calcium (Ca2+) channels at the base of these hair cells (Castro et al., 2003). This triggers an inward movement of calcium into the hair cells, which in turn initiates the release of glutamate (a fast excitatory neurotransmitter) from the synaptic vessels. The cochlear nerve terminals pick up the neurotransmitter and generate action potentials that travel to the cochlear nuclear complex located at the pontomedullary junction.
COCHLEAR AMPLIFIER
Basilar membrane movement provides passive analysis of sound. The OHC help provide active analysis. In other words, OHC serve to alter cochlear mechanicsāthat is, basilar membrane movement.
OHC have been found to contain contractile proteins. Stimulation of the cilia of the OHC (shearing action of the cilia) allows the endolymphic potential to pass through and cause the OHCs to contract or oscillate. The stimulated OHCs oscillate at the same frequency of the stimulus. This oscillation produces a receptor potential called the cochlear microphonic. This potential mimics the waveform of the stimulus. Because the cilia of the OHC make direct contact with the tectorial membrane, the contractile property of the OHC causes BM movement to be more finely tuned. The contractile properties of the OHC also serve to increase the sensitivity of the ear as a cochlear amplifier. OHC oscillation increases the motion in the cochlea, allowing the tectorial membrane to stimulate (or shear) the cilia of the IHCāthe true sensory cells of hearing. OHC contraction (and thus amplification) is dependent on the intensity of the stimulus. OHC participation is greater at low intensities and less at high intensities.
NEURAL CODING OF AUDITORY INFORMATION
The transmission of auditory information from the cochlea to the central auditory nervous system is coded so that all its elementsātiming, intensity, frequency, and othersāare fully retained. The exact mechanism and format for coding acoustic information are not completely understood. However, it is likely that this information is coded in a variety of ways to ensure a degree of redundancy. For example, cochlear representation of frequency is based on the stimulation of hair cells at a specific region along the basilar membrane. Although cochlea neural units can be stimulated by a wide variety of sound frequencies, they ...