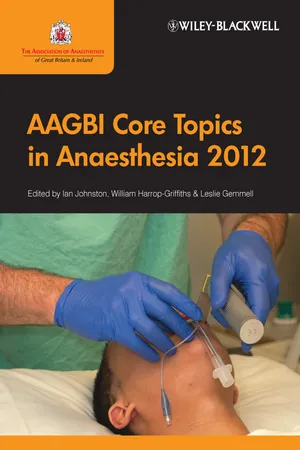
eBook - ePub
AAGBI Core Topics in Anaesthesia 2012
Ian Johnston, William Harrop-Griffiths, Leslie Gemmell, Ian Johnston, Leslie Gemmell, William Harrop-Griffiths
This is a test
Share book
- English
- ePUB (mobile friendly)
- Available on iOS & Android
eBook - ePub
AAGBI Core Topics in Anaesthesia 2012
Ian Johnston, William Harrop-Griffiths, Leslie Gemmell, Ian Johnston, Leslie Gemmell, William Harrop-Griffiths
Book details
Book preview
Table of contents
Citations
About This Book
Based on the Association of Anesthetists of Great Britain and Ireland's (AAGBI) continuing education lecture series, this clinical-oriented book covers the latest developments in research and the clinical application to anesthesia and pain control.
Frequently asked questions
How do I cancel my subscription?
Can/how do I download books?
At the moment all of our mobile-responsive ePub books are available to download via the app. Most of our PDFs are also available to download and we're working on making the final remaining ones downloadable now. Learn more here.
What is the difference between the pricing plans?
Both plans give you full access to the library and all of Perlego’s features. The only differences are the price and subscription period: With the annual plan you’ll save around 30% compared to 12 months on the monthly plan.
What is Perlego?
We are an online textbook subscription service, where you can get access to an entire online library for less than the price of a single book per month. With over 1 million books across 1000+ topics, we’ve got you covered! Learn more here.
Do you support text-to-speech?
Look out for the read-aloud symbol on your next book to see if you can listen to it. The read-aloud tool reads text aloud for you, highlighting the text as it is being read. You can pause it, speed it up and slow it down. Learn more here.
Is AAGBI Core Topics in Anaesthesia 2012 an online PDF/ePUB?
Yes, you can access AAGBI Core Topics in Anaesthesia 2012 by Ian Johnston, William Harrop-Griffiths, Leslie Gemmell, Ian Johnston, Leslie Gemmell, William Harrop-Griffiths in PDF and/or ePUB format, as well as other popular books in Médecine & Soins infirmiers. We have over one million books available in our catalogue for you to explore.
Information
CHAPTER 1
The Physics of Ultrasound
Key points
- Ultrasound is a high-frequency pressure energy wave transmitted longitudinally through the soft tissues of the body.
- Advances in computer technology have made medical ultrasound possible by processing millions of signals every second.
- Ultrasound makes it possible to examine most of the tissues of the body safely and easily.
- The pressure, energy and heating effects of clinical ultrasound devices have not been shown to damage normal biological tissues.
- The ultrasound wave must be reflected off a tissue interface at right angles. This means that a combination of good hand–eye coordination and correct positioning of the probe is the basis of a good image.
- Images are presented as patterns on a greyscale monitor. Pattern recognition is therefore the basis of the interpretation of these images.
- The B or brightness mode gives a greyscale image that is distorted because of a loss of reflected echoes by scatter and refraction.
- Doppler shift is caused by a change in wavelength when fluid such as blood is moving towards or away from the ultrasound wave.
This chapter aims to give an introduction to the basic physics of ultrasound in order to allow the reader to understand how images are produced and hence how to obtain the best images when using ultrasound. Clinical ultrasound devices simultaneously produce and transmit multiple pressure waves, and receive and rapidly interpret the many attenuated, returning pressure wave signals. The pressure wave signals are converted into electrical signals. The production of an image in real time by a portable scanner has only become possible with the development of the microprocessor chip. A modern ultrasound device has a lot of computing power with many microprocessors performing many billion operations per second, which makes it possible to build up complicated images in real time. In the near future, smaller processors will enable more precise images to be created on smaller, lighter and cheaper devices.
Sound energy
Sound is energy in the form of a pressure wave. This mechanical energy displaces molecules that press against adjacent molecules and pass the energy on in waves, while the first molecules to be compressed together return to their original configuration. Ultrasound waves travel in a longitudinal direction, rather like a piston pumping in and out, producing a series of high pressure, unidirectional waves in front of the piston. The effect can be visualised as the energy of the wind blowing through a cornfield. The corn bends over but returns to the upright as the wind blows through the field. The pressure recorded in ultrasound waves can be as high as 10 times atmospheric pressure but it is a transient change. A sound wave needs molecules to pass the wave on so it cannot travel through a vacuum, and medical ultrasound does not pass air so, for instance, the lung will appear black unless there is an effusion or consolidation within the lung. The principle behind obtaining information using ultrasound is to send a short burst of pressure waves into the tissue, wait for the initial wave to bounce back from a number of tissue interfaces, and then analyse the waves that return. This can be understood with reference to the early application of sound waves in Sonar. A single wave of sound is sent out from a boat into the water and the time taken for the echo to return is measured. Knowing the speed of sound in water and the time taken for the sound to return, the distance travelled by the sound can be calculated; the point at which the sound is reflected is half this distance.
Generation of the ultrasound wave
In order to build up an image of the tissues, it is necessary to have many sound waves transmitted from a series of points fixed in relationship to each other. This is made possible with a line or array of transducers in the probe head. The probe is the most delicate part of the device and a typical array contains 128–256 transducer elements in a wide aperture device. There are matrix arrays now on the market with up to 2400 transducer elements. Each transducer has a dual function. It both creates and sends out a short burst of ultrasound pressure waves, and is then silent until it detects those waves returning. This is referred to as pulsed ultrasound. Small groups of transducers are activated in turn to emit at the same time followed by another group. In this way some part of the probe head is always emitting and some part is always receiving. In order to build up an image, it is necessary to emit and receive many signals in a short period of time. This is made possible by the use of materials that exhibit the piezoelectric effect. Pierre and Jacques Curie found that a crystal of quartz changes shape when an electrical charge or voltage is applied across it and, conversely, when pressure is applied to the crystal, it changes its shape and an electrical charge is created. This two-way process of an electrical charge causing a change in shape that produces a pressure wave, and pressure causing a change in shape that creates an electrical charge, is known as the piezoelectric effect. The emitted ultrasound signal is produced by applying an electrical charge to the transducer and the returning pressure wave distorts the transducer to create an electrical charge that can then be processed. The sensitivity of the transducer has been increased by replacing quartz crystals with materials such as lead zirconate titanate (PZT) mixed with epoxy, and polyvinylidene difluoride (PVDF). The transducer is made by heating powdered PZT to above its Curie temperature (365°C for PZT), sometimes mixed with epoxy to make a composite ceramic. While the crystals are hot and mobile a high voltage current is applied across the transducer to polarise the crystals so that they line up to give a maximum response to changes when an external electrical charge or pressure is applied. The voltage is maintained until the mixture cools and solidifies so that the alignment of the crystals is preserved in the solid state.
Refinements in transducer production
Each transducer has a particular frequency at which it will convert electrical energy into sound waves and vice versa. This is the resonance frequency, which is mostly dependent on the thickness of the transducer element: the thinner the element, the higher the frequency. In practice, the emitted wave is not a single wavelength but a spectrum of frequencies. These are produced by forming multiple matching layers on the surface of the element. These layers also provide good sound transmission between the transducer and the soft tissues over a range of frequencies. Modern transducers are constructed of piezoelectric materials that have similar acoustic impedances to that of soft tissue so that sound is not lost by reflection at the soft tissue-transducer interface.
The frequency, wavelength and speed of sound waves
A sound wave has characteristics of frequency, wavelength and speed. The frequency and speed of the sound wave determine the type of substance through which it will travel.
Frequency
The frequency (f) is the number of high-pressure waves in one second. The time taken from one high pressure to the next high pressure is known as the period (T). Therefore, T = 1/f
Frequency is quoted in Hz where 1 Hz = 1 cycle.s−1. Low-frequency sound waves or seismic waves are transmitted through dense materials such as the earth. Sound waves that are below the audible range are called infrasonic. Sound that is heard has a frequency in the range 20 Hz to 20 kHz. This is called the audible frequency range. Audible sound waves are transmitted through air at a speed of 330 m.s−1. Ultrasound has a frequency above the audible range at 20 kHz. Clinical ultrasound is in the range of 2–15 MHz. This is transmitted well through the soft tissues of the body. Air-filled cavities and solid material such as bone and metal needles will not transmit this frequency of sound, so the lung and intracranial structures cannot be examined, except in babies when ultrasound can be focused through the fontanelles.
Wavelength
The wavelength (λ) is the distance between two high-pressure peaks in the sound wave. The wavelength is the speed of the sound wave divided by the frequency of the high-pressure components, hence λ = c/f. The wavelength is important for spatial resolution. This is the ability to identify two objects when one is deeper than the other (longitudinal spatial resolution) or side-by-side (axial spatial resolution) but only separated by a short distance. In soft tissues, wavelengths of about 1 mm are used (at 2 MHz the wavelength is 0.77 mm). A higher frequency produces a shorter wavelength. The width of the wave is about the same as the wavelength, so the width of the sound wave becomes smaller as the frequency increases. If the object presents a surface area that is less than the width of the sound wave, such as a red blood cell, the wave will not be reflected at 180°.
Speed
The speed of the sound wave is determined by the nature of the substance or organ it passes through. The speed c = √B/ρ, where B is the stiffness or bulk modulus and ρ is the density of the tissue or organ. The optimum speeds of sound for the different soft tissues of the body are:
| 1580 m.s−1 1575 m.s−1 1570 m.s−1 1480 m.s−1 1430 m.s−1 |
A convention exists in which manufacturers of medical ultrasound devices all use the same sound wave speed to allow images to be comparable. This speed is 1540 m.s−1. Therefore, clinical ultrasound will not pass through materials that require a much higher or lower speed for wave transmission:
| 330 m.s−1 1240 m.s−1 2800 m.s−1 4080 m... |