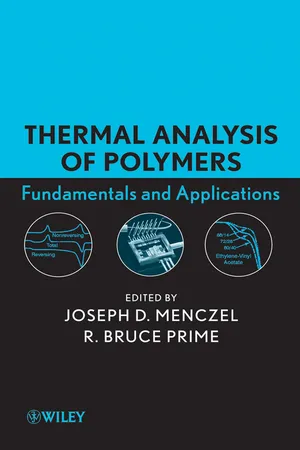
eBook - ePub
Thermal Analysis of Polymers
Fundamentals and Applications
This is a test
- English
- ePUB (mobile friendly)
- Available on iOS & Android
eBook - ePub
Thermal Analysis of Polymers
Fundamentals and Applications
Book details
Book preview
Table of contents
Citations
About This Book
- Presents a solid introduction to thermal analysis, methods, instrumentation, calibration, and application along with the necessary theoretical background.
- Useful to chemists, physicists, materials scientists, and engineers who are new to thermal analysis techniques, and to existing users of thermal analysis who wish expand their experience to new techniques and applications
- Topics covered include Differential Scanning Calorimetry and Differential Thermal Analysis (DSC/DTA), Thermogravimetry, Thermomechanical Analysis and Dilatometry, Dynamic Mechanical Analysis, Micro-Thermal Analysis, Hot Stage Microscopy, and Instrumentation.
- Written by experts in the various areas of thermal analysis
- Relevant and detailed experiments and examples follow each chapter.
Frequently asked questions
At the moment all of our mobile-responsive ePub books are available to download via the app. Most of our PDFs are also available to download and we're working on making the final remaining ones downloadable now. Learn more here.
Both plans give you full access to the library and all of Perlegoâs features. The only differences are the price and subscription period: With the annual plan youâll save around 30% compared to 12 months on the monthly plan.
We are an online textbook subscription service, where you can get access to an entire online library for less than the price of a single book per month. With over 1 million books across 1000+ topics, weâve got you covered! Learn more here.
Look out for the read-aloud symbol on your next book to see if you can listen to it. The read-aloud tool reads text aloud for you, highlighting the text as it is being read. You can pause it, speed it up and slow it down. Learn more here.
Yes, you can access Thermal Analysis of Polymers by Joseph D. Menczel, R. Bruce Prime, Joseph D. Menczel, R. Bruce Prime in PDF and/or ePUB format, as well as other popular books in Physical Sciences & Analytic Chemistry. We have over one million books available in our catalogue for you to explore.
Information
CHAPTER 1
INTRODUCTION
Thermal analysis (TA) comprises a family of measuring techniques that share a common feature; they measure a materialâs response to being heated or cooled (or, in some cases, held isothermally). The goal is to establish a connection between temperature and specific physical properties of materials. The most popular techniques are those that are the subject of this book, namely differential scanning calorimetry (DSC), thermogravimetric analysis (TGA), thermomechanical analysis (TMA), dynamic mechanical analysis (DMA), dielectric analysis (DEA), and micro/nano-thermal analysis (Îź/n-TA).
This book deals almost exclusively with studying polymers, by far the widest application of thermal analysis. In this area, TA is used not only for measuring the actual physical properties of materials but also for clarifying their thermal and mechanical histories, for characterizing and designing processes used in their manufacture, and for estimating their lifetimes in various environments. For these reasons, thermal analysis instruments are routinely used in laboratories of the plastics industry and other industries where polymers and plastics are being manufactured or developed. Thus, thermal analysis is one of the most important research and quality control methods in the development and manufacture of polymeric materials as well as in industries that incorporate these materials into their products.
Not withstanding its importance, educational programs in thermal analysis at universities and colleges are almost nonexistent; certainly they are not systematic. Thermal analysis training in the United States is for the most part limited to short courses, such as the short course at the annual meeting of the North American Thermal Analysis Society (NATAS) and earlier the short course at the Eastern Analytical Symposium. Our goal was to write a book that could be used as a text or reference to accompany thermal analysis courses and that would enable both beginners and experienced practitioners to do some self-education. This book is for experimenters at all levels that addresses both the fundamentals of the thermal analysis techniques as well as the practical issues associated with the running of experiments and interpretation of the results. Several examples are given where thermal analysis played a key role in solving a practical problem, and they are presented in a manner that will allow readers to apply the lessons to their own problems.
This book is organized by measuring techniques rather than by material classification. These techniques all follow the change of specific physical properties as the temperature and possibly atmosphere are controlled. Table 1.1 indicates the classification of the more common techniques by the physical property measured.
Most thermal analysis studies today are conducted with commercial instruments. Manufactures have striven to provide complete âsystemsâ capable of a wide range of analyses and frequently sharing modular components. Naturally, this is a market-driven phenomenon, and the current driving forces are speed, miniaturization, and automation. The goals of a modern industrial quality control facility, a state-of-the-art research institution, or those of a teaching laboratory are quite different. This difference leads to a broad spectrum of available instrumentation in terms of ultimate capabilities, simplicity, and cost.
Commercial thermal analysis instrumentation is relatively new, a product of the last four decades or so. Mass production of TA instruments started in the early 1950s. From then to the 1970s, several major TA instruments were marketed, and some of them are still manufactured even today. This was the time when the two types of DSC (power compensation and heat flux) appeared, and the principle of the measurements is still the same today.
TABLE 1.1. The Most Important General Methods and Techniques of Thermal Analysis
General Method | Acronym | Property Measured |
Differential Scanning Calorimetry | DSC | ÎT, differential power input |
Differential Thermal Analysis | DTA | ÎT |
Thermogravimetry or Thermogravimetric Analysis |
TG or TGA | Mass |
Thermomechanical Analysis, Thermodilatometry |
TMA, TD | Length or volume |
Dynamic Mechancal Analysis | DMA | Viscoelastic properties |
Dielectric Analysis | DEA | Dielectric properties |
Micro/Nano-Thermal Analysis | Îź/n-TA | Penetration, ÎT |
A gigantic leap forward in the evolution of scientific instrumentation and data analysis took place with the advent of the digital computer. The replacement of such things as chart recorders and analog computers has dramatically improved our ability to control, measure, and evaluate experiments precisely. This process in TA introduced revolutionary changes. No longer was there a need to rerun the measurement if the sensitivity was not properly adjusted, the accuracy of the measurements dramatically increased, the time necessary for data evaluation decreased significantly, and many measurements became automated. The use of autosamplers doubled or tripled the throughput of the instruments, allowing them to run day and night. To complement the autosamplers, cooling units can be turned on and off at a pre-programmed time in the absence of the operator.
At the same time, problems developed because of certain software issues. When software is automatically capable of performing calculations, the operator often tends to be lazy and fails to learn the theoretical basis of the measurement and the calculation. Although it is certainly not the intention of any instrument manufacturer or software purveyor to deceive or mislead the user, slavish reliance on software without adequate comprehension can be dangerous. As an example, the quest for nice-looking plots can lead to excessive smoothing or dampening of the results, with the possible consequence of missing meaningful, even critical, subtle events. Another negative aspect of blind software use is the question of significant figures. Modern scientific literature is replete with insignificant figures. The ability of a computer to generate an unending series of digits is no indication or justification of their relevance. And although the manufacturers often provide the option to change the number of displayed digits, with the rush in modern laboratories, operators often fail to make this change. But eventually, it is incumbent on investigators to evaluate the results of their analyses, with regard to both the significance of the numbers and their conclusions.
The number of instrument manufacturers decreased somewhat during the last decade or so, but there are still a significant number on the market. Today it seems that most of these corporations will survive. Although some are highly specialized, almost all thermal analysis instrument companies produce one or more DSCs. As mentioned, popularity has its disadvantages: DSC and TGA are the two most popular TA techniques, and these are the ones that many operators routinely use, often without the necessary theoretical knowledge. This lack of understanding creates such an absurd situation that the essence of DSC measurements is reduced to recording a melting peak, whereas in TGA, all they look for is the start of the mass loss. Similarly, TMA is often reduced to looking for the shift in the slope of the dimension versus temperature curves to measure Tg. Interestingly, experimenters who use the relaxation techniques (DMA and DEA), and Îź/n-TA, tend to rely more on theory and do fewer simple repetitive measurements.
Temperature in thermal analysis is the most important parameter. The strict definition of TA stipulates a programmed (i.e., time- or property-dependent) temperature. From the standpoint of instrumentation and methodology, however, isothermal measurements are included. Some applications concerning kinetics, as an example, involve a series of isothermal measurements at different temperatures or measuring an isothermal induction time to reaction. Other isothermal techniques may involve time to ignition and changes in the measured property with a changing atmosphere or force applied to the sample. Temperature is conveniently and most often measured by thermocouples, either individually or coupled in series as a thermopile to increase the sensitivity and/or to integrate the measurement over a greater volume. Some instruments use platinum resistance thermometers. Optical pyrometry has been applied in rare instances. These latter two methods are the methods of choice, depending on the specific temperature, as set forth in the definition of the International Temperature Scale. Regardless of the particular sensor used, calibration of the temperature is generally dependent on the specific technique and will be discussed with each class of instrumentation. Careful consideration must always be given when equating sensor temperature with actual sample temperature. Depending on the enthalpy of the processes or reactions occurring, thought must also be given to equating a âbulkâ sample temperature with that at the interface where the actual reaction may be taking place. Such considerations are particularly important for meaningful kinetic analyses.
Thermal analysis can be used in a variety of combinations. The most common combinations all share the same sample as well as thermal environment. A key distinction is made between true simultaneous methods like TGA/DTA and TGA/DSC, in which there is no time delay between the measurements, and near-simultaneous measurements like TGA/MS and TGA/ FTIR, in which the time delay is small between the mass loss and the respective gas detector. Such combined techniques not only represent a saving in time, but also they help to alleviate or minimize uncertainties in the comparison of such results. And TGA/MS and TGA/IR can be instrumental in identifying complex processes that involve mass loss. Although combined instruments are not described in this book in detail, some examples are given in the TGA chapter.
Unfortunately, the proper selection of the run parameters is often ignored in thermal analysis measurements, even though it is a critical part. Of all the run parameters, the sample mass, the ramp rate, and the purge gas are the most important. The sample size and its physical shape play a significant role in the results. The proper sample size and the heating rate are interconnected because in several techniques faster rates require smaller samples and substantially improved conditions for rapid thermal transport between the sample and its controlled environment. Therefore, compromises are necessary between sample size and heating and cooling rates. And thermal conductivity and flow of the atmosphere thus become significant factors. Transport conditions may change substantially with temperature as the nature of the thermal path and the relative roles of conduction, convection, and radiation are altered.
Traditionally, simple combinations of linear heating or cooling rates and isothermal segments have been employed. Modern methods, however, frequently impose cyclic temperature programs coupled with Fourier analyses to achieve particular advantages and added information. These approaches are referred to as modulated techniques, and temperature is the most commonly modulated parameter. Note that in DMA, stress or strain is the modulated parameter and that in DEA, the electric field is modulated, but in modulated temperature DSC and modulated temperature TMA, it is the temperature that is modulated.
Often interaction of the sample with its atmosphere is important, for example, in oxidation/reduction reactions or catalytic processes. Reversible processes will be influenced by product accumulations, and hence, the ability of the flowing atmosphere to purge these volatile products becomes important. A common example of such a reversible process is dehydration or solvent removal. Clearly the degree of exposure of the sample to its atmosphere then becomes a factor. Deliberate modifications of the sample holder or compartment are made to control these interactions. Maximum exposure can be attained with the sample in a thin bed with the atmosphere flowing over or even percolating through it. Minimum exposure can be achieved using sealed sample containers or ones with a small orifice to alleviate the changes of pressure resulting from temperature changes and/or reactions with the gas phase. Diffusion of species into and out of the sample also needs to be considered. For example, in oxidative processes, diffusion of oxygen into the sample becomes important, and in mass loss processes, the volatile products need to diffuse to the surface where evaporation occurs. In these cases, sample size and shape, e.g., surface-to-volume ratios, may influence the results.
The above considerations all dictate that the sample size and form can be a significant factor in the effort to achieve the desired analysis and its reliability. The ability to impose a rapidly changing thermal environment on the sample may be necessary to simulate the true process conditions properly or simply to obtain the results more quickly. As mentioned, this necessity dictates a small sample in order to follow the temperature program, but this in turn demands a representative sample. Thus, it may be difficult or impossible to achieve for a very small sample of some materials. Composites, blends, and naturally occurring materials may lack the necessary homogeneity. Reproducibility of the measurements or even other analytical data is needed to assure that the sample is indeed representative.
Even though the inhomogeneity of the sample at fast heating rates can be compensated for with a smaller sample size, there are time-dependent phenomena in most thermal analysis techniques. One can change the heating rate and ensure an acceptable temperature gradient in the sample, and different physical processes may and will take place at different heating rates as demonstrated in Chapters II and III (DSC and TGA). Thus, the selection of a proper ramp rate is important, and just by changing the sample size, one cannot compensate for all the variabilities in the sample.
A word about mass is appropriate. The International Union of Pure and Applied Chemistry (IUPAC) and the International Confederation of Thermal Analysis and Calorimetry (ICTAC) have determined that the property measured by TGA should be referred to as âmassâ and not as âweight.â Although some figures are reproduced with their original ordinates, which may be weight or weight percent, we adhere to this terminology and refer to sample mass, mass percent, and mass loss. It is still correct to refer to the weighing of samples and to standard reference weights.
CHAPTER 2
DIFFERENTIAL SCANNING CALORIMETRY (DSC)
2.1. INTRODUCTION
Differential scanning calorimetry (DSC) is the most popular thermal analysis technique, the âworkhorseâ of thermal analysis. This is a relatively new technique; its name has existed since 1963, when Perkin-Elmer marketed their DSC-1, the first DSC. The term DSC simply implies that during a linear temperature ramp, quantitative calorimetric information can be obtained on the sample. According to the ASTM standard E473, DSC is a technique in which the heat flow rate difference into a substance and a reference is measured as a function of temperature, while the sample is subjected to a controlled temperature program. As will be seen from this chapter, the expression âDSCâ refers to two similar but somewhat different thermal analysis techniques. It is a common feature of these techniques that the various characteristic temperatures, the heat capacity, the melting and crystallization temperatures, and the heat of fusion, as well as the various thermal parameters of chemical reactions, can be determined at constant heating or cooling rates. It is important to note that the acronym DSC has two meanings: (1) an abbreviation of the technique (i.e., differential scanning calorimetry) and (2) the measuring device (differential scanning calorimeter).
Since the 1960s the application of DSC grew considerably, and today the number of publications that report DSC must amount to more than 100,000 annually.
One of these techniques that brought into science the name DSC, called today power compensation DSC, was created by Gray and OâNeil at the Perkin-Elmer Corporation in 1963. The other technique grew out of differential thermal analysis (DTA), and is called heat flux DSC. Differential thermal analysis itself originates from the works of Le Chatelier (1887), Roberts-Austen (1899), and Kurnakov (1904) (see Wunderlich, 1990). It needs to be emphasized that both of these techniques give similar results, but of course, they both have their advantages and disadvantages.
The major applications of the DSC technique are in the polymer and pharmaceutical fields, but inorganic and organic chemistry have also benefited significantly from the existence of DSC. Among the applications of DSC we need to mention the easy and fast determination of the glass transition temperature, the heat capacity jump at the glass transition, melting and crystallization temperatures, heat of fusion, heat of reactions, very fast purity determination, fast heat capacity measurements, characterization of thermosets, and measurements of liquid crystal transitions. Kinetic evaluation of chemical reactions, such as cure, thermal and thermooxidative degradation is often possible. Also, the kinetics of polymer crystallization can be evaluated. Lately, among the newest users of DSC we can list the food industry and biotechnology. Sometimes, specific DSC instruments are developed for these consumers.
DSC is extremely useful when only a limited amount of sample is available, since only milligram qu...
Table of contents
- COVER PAGE
- CONTENTS
- TITLE PAGE
- COPYRIGHT PAGE
- PREFACE
- CHAPTER 1: INTRODUCTION
- CHAPTER 2: Differential Scanning Calorimetry (DSC)
- CHAPTER 3: THERMOGRAVIMETRIC ANALYSIS (TGA)
- CHAPTER 4: THERMOMECHANICAL ANALYSIS (TMA) AND THERMODILATOMETRY (TD)
- CHAPTER 5: DYNAMIC MECHANICAL ANALYSIS (DMA)
- CHAPTER 6: DIELECTRIC ANALYSIS (DEA)
- CHAPTER 7: MICRO- AND NANOSCALE LOCAL THERMAL ANALYSIS
- INDEX