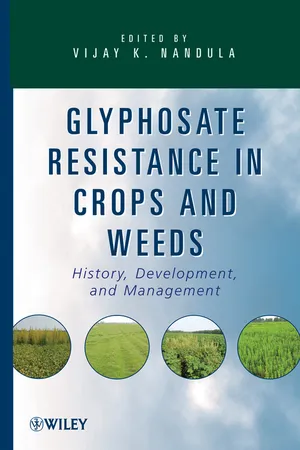
eBook - ePub
Glyphosate Resistance in Crops and Weeds
History, Development, and Management
This is a test
- English
- ePUB (mobile friendly)
- Available on iOS & Android
eBook - ePub
Glyphosate Resistance in Crops and Weeds
History, Development, and Management
Book details
Book preview
Table of contents
Citations
About This Book
New technologies are becoming available for managing glyphosate resistant (GR) weeds and reducing their spread. GR crop technology has revolutionized crop production in the developed world and the benefits are gradually spilling over to the developing world. In order to sustain an effective, environmentally safe herbicide such as glyphosate and the GR crop technology well in to the future, it is imperative that the issue of GR weeds be comprehensively understood. This book provides such an essential, up-to-date source of information on glyphosate resistance for researchers, extension workers, land managers, government personnel, and other decision makers.
- Provides comprehensive coverage of the intensely studied topic of glyphosate resistant (GR) in crops
- Details the development of glyphosate resistance and how to detect and manage the problem in crops
- Helps standardize global approaches to glyphosate resistance
- Encompasses interdisciplinary approaches in chemistry, weed science, biochemistry, plant physiology, plant biotechnology, genetics, ecology
- Includes a chapter on economic analysis of GR impact on crops
Frequently asked questions
At the moment all of our mobile-responsive ePub books are available to download via the app. Most of our PDFs are also available to download and we're working on making the final remaining ones downloadable now. Learn more here.
Both plans give you full access to the library and all of Perlegoās features. The only differences are the price and subscription period: With the annual plan youāll save around 30% compared to 12 months on the monthly plan.
We are an online textbook subscription service, where you can get access to an entire online library for less than the price of a single book per month. With over 1 million books across 1000+ topics, weāve got you covered! Learn more here.
Look out for the read-aloud symbol on your next book to see if you can listen to it. The read-aloud tool reads text aloud for you, highlighting the text as it is being read. You can pause it, speed it up and slow it down. Learn more here.
Yes, you can access Glyphosate Resistance in Crops and Weeds by Vijay K. Nandula, Vijay K. Nandula in PDF and/or ePUB format, as well as other popular books in Physical Sciences & Organic Chemistry. We have over one million books available in our catalogue for you to explore.
Information
1
GLYPHOSATE: DISCOVERY, DEVELOPMENT, APPLICATIONS, AND PROPERTIES
1.1 HISTORICAL PERSPECTIVE AND MODE OF ACTION
N-(phosphonomethyl)glycine (glyphosate) is a phosphonomethyl derivative of the amino acid glycine. Glyphosate is a white and odorless crystalline solid comprised of one basic amino function and three ionizable acidic sites (Fig. 1.1). Glyphosate was actually invented in 1950 by a Swiss chemist, Dr. Henri Martin, who worked for the small pharmaceutical company, Cilag (Franz et al. 1997). The product had no pharmaceutical application and was never reported in literature. In 1959, Cilag was acquired by Johnson and Johnson, which sold its research samples, including glyphosate, to Aldrich Chemical. Aldrich sold small amounts of the compound to several companies in the 1960s for undisclosed purposes, but no claims of biological activity were ever reported. In its Inorganic Division, Monsanto was developing compounds as potential water-softening agents and over 100 related aminomethylphosphonic acid (AMPA) analogs were synthesized. When these compounds were tested as herbicides by Dr. Phil Hamm, two showed some herbicidal activity on perennial weeds. However, the unit activity was too low to be a commercial herbicide.
Figure 1.1. The structure of glyphosate.
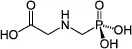
Dr. Hamm enlisted the efforts of Monsanto chemist Dr. John Franz. He repeatedly told Dr. Franz that āhe just wanted something five times as strong ⦠thatās all.ā āHe convinced me to take a shot at making analogs and derivatives,ā recalled Dr. Franz. āThat didnāt yield anything, and I was ready to drop the project. But then I began trying to figure out the peculiarities of those two compounds, and I wondered if they might metabolize differently in the plants than the others ⦠I began to write out metabolites ⦠you could write a list of about seven or eight ⦠it involved completely new chemistry. Glyphosate was the third one I madeā (Halter 2007). Glyphosate was first synthesized by Monsanto in May 1970 and was tested in the greenhouse in July of that year. The molecule advanced through the greenhouse screens and field testing system rapidly and was first introduced as RoundupĀ® herbicide by Monsanto Company (St. Louis, MO) (Baird et al. 1971).
Glyphosate inhibits the enzyme 5-enolpyruvylshikimate-3-phosphate synthase (EPSPS) (Amrhein et al. 1980), which is present in plants, fungi, and bacteria, but not in animals (Kishore and Shah 1988). The enzyme catalyzes the transfer of the enolpyruvyl moiety of phosphoenolpyruvate (PEP) to shikimate-3-phosphate (S3P). This is a key step in the synthesis of aromatic amino acids, and ultimately, hormones and other critical plant metabolites. The active site of the EPSPS enzyme in higher plants is very highly conserved (CaJacob et al. 2003). The mechanism of inhibition is also unique in that the binding site for glyphosate is reported to closely overlap with the binding site of PEP (Franz et al. 1997). A diagram of the shikimate pathway and glyphosateās inhibition site is shown in Figure 1.2. No other mode of action for glyphosate has been observed even when very high doses are applied to glyphosate-resistant (GR) soybean and canola (Nandula et al. 2007).
Figure 1.2. The site of inhibition of glyphosate from Dill (2005).
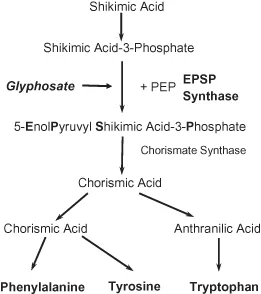
Glyphosate is currently labeled for use in over 130 countries, and current global volume is estimated to be approximately 600 kilotons annually (Research and Markets 2008). The current U.S. glyphosate label of Monsanto Agricultural Herbicides lists over 100 annual broad-leaved and grass species controlled. In addition, over 60 perennial weed species are also included on the label as of the writing of this chapter. It is the broad spectrum perennial weed control that makes glyphosate a very effective product. The ability of the product to translocate to growing meristematic tissues and inhibit an enzymatic process present in plants allows applicators to control underground meristems, corms, rhizomes, and other potential vegetative structures, which regenerate when only upper vegetative material is killed.
Because of its unique properties, glyphosate was initially utilized to control perennial weeds on ditch banks, in right of ways, and fallow fields. However, because it also killed crops, its uses in mainstream agriculture were limited until the use of minimum and no-till practices began to evolve. Spraying glyphosate to control weeds prior to planting allows growers to substitute chemical weed control with light-duty spray equipment for tillage. This practice saves fuel, preserves soil from erosion, and allows better water permeation into the soil (Dill 2005). Conservation tillage practices have continued to grow with the introduction of GR crops (Dill et al. 2008).
1.2 UPTAKE AND TRANSLOCATION OF GLYPHOSATE
The herbicidal efficacy of glyphosate is strictly dependent on the dose of glyphosate delivered to the symplastic or living portion of the plant. Since glyphosate was first announced (Baird et al. 1971) as a broad spectrum herbicide (and before the evolution of GR weeds), it could be said that all plants could be controlled given delivery of the appropriate dose of glyphosate. The delivery of this efficacious dose has continually been the topic of investigation now for almost 40 years with at least 40 individual weed species now studied in detail to determine the efficiency of uptake and the extent of translocation. The corollary science of pesticide application is an extensive area covering the physics of spray application and the reader is directed to a standard text (Monaco et al. 2002), while here we focus on uptake and translocation.
The first uptake efficiency and translocation studies of 14C-glyphosate (Sprankle et al. 1973) characterized the principal features of glyphosate that we know today: phloem transport and consequent delivery to meristematic growing points in the roots and vegetation. The phloem movement of glyphosate intimately linked the efficiency of translocation to plant health and developmental stage, which are tied to environmental conditions. The early work is well summarized in the book The Herbicide Glyphosate (Caseley and Coupland 1985). The discovery of the mode of action of glyphosate to be the inhibition of EPSPS (Steinrucken and Amrhein 1980) was largely due to the very rapid large accumulation of shikimic acid (Amrhein et al. 1980), which now routinely serves as a means to measure glyphosate toxicity (Singh and Shaner 1998).
Uptake and translocation studies are two different types of studies that are often combined as one to the detriment of both. Uptake studies should focus on the drop size and solute concentrations (and not really the total dose), whereas translocation studies require precise dose amounts so that distribution ratios can be calculated. There is a conundrum in uptake studies between volume and concentration when trying to deliver the desired dose. It is virtually impossible to deliver by hand application the droplets dictated by typical carrier volumes; the drops are just too small and too numerous. Consequently, the experimental dose is usually applied in a much smaller volume and/or much larger drop, dramatically distorting the concentration ratios of herbicide : surfactant: carrier volume ruining the lessons to be learned about the efficiency of spray solution penetration. Understanding the impact of spray solution composition on the efficiency of glyphosate penetrating the cuticle to the apoplast and the stepwise entry into the symplast where phloem transport can occur is critical to optimizing herbicide formulation. Normally, the amount of glyphosate āinsideā the leaf or not removed by washing is considered the efficiency of uptake. Uptake is dependent on several interdependent factors: droplet size and droplet spread, cuticle composition and thickness, surfactant type and concentration, ionic strength and salt concentration, humidity, and, most importantly, glyphosate concentration. Because of the critical linkages between these factors, the most informative uptake studies are done with a sprayed application using a standard field nozzle and carrier (Feng et al. 2000; Prasad 1989, 1992). However, it is extremely difficult to deliver a precise dose due to the practical problems of leaf intercept of a spray application, and so the leaf intercept efficiency must be included. The interaction of drop size, surfactant, and herbicide concentration does impact the leaf surface cytology and can be correlated to efficiency of uptake (Feng et al. 1998, 2000). The cytotoxic damage caused by the excess surfactant/cuticle surface area provided by a large drop quickly ākillsā the loading site for translocation and prematurely stops phloem loading. The exact correlation of drop size and concentration to penetration was determined by using a droplet generator (Prasad and Cadogan 1992). The herbicide concentration in very small droplets did overcome the drop-size factors, and the smaller droplets had minimal negative effect on epidermal cytology (Ryerse et al. 2004), thereby, avoiding the inhibition of transport caused by too much local cell damage (often seen in hand-applied large drops). The concept that small spray droplets do not actually dry but soak into the leaf was shown by coapplication with heavy water (deuterium oxide, D2O), indicating that the surfactant forms channels to allow the herbicide to penetrate the cuticle as measured by the appearance of D2O in the leaf (Feng et al. 1999). Spray applications on GR corn then allowed the separation of local droplet-herbicide toxicity from droplet-surfactant injury related to drop size to show that large drops, while being retained less efficiently, were more efficient at loading glyphosate and allowing improved translocation. Consequently, studies that spray 14C-glyphosate provide the best means to mimic field conditions and simultaneously understand the formulated droplet uptake characteristics (Feng and Chiu 2005; Feng et al. 2000, 2003b).
Translocation efficiency is dramatically affected by the self-limitation feature of glyphosate toxicity (vide infra) creating another paradox, optimizing translocation (improving with time) with increasing toxic effect (increasing with time). The negative effects on apical meristems with a small dose of glyphosate are readily accounted for by the observation that individual plant tissues have different sensitivities to glyphosate (Feng et al. 2003a). This toxicity affects the overall glyphosate efficiency and distribution pattern to sink tissues. Dewey (1981) noted that glyphosate easily loaded the phloem, moved from source to sink, and did not usually leave the symplastic assimilant flow. Gougler and Geiger (1981) used a sugar beet model system to demonstrate that glyphosate loads the phloem passively, and this result holds true as no significant active transport of glyphosate has ever been measured. They subsequently showed that reductions in photosynthesis resulted directly in limiting glyphosate translocation (Geiger et al. 1986) and further that glyphosate created a self-limitation of translocation due to its toxicity shutting down photosynthesis and sucrose metabolism (Geiger and Bestman 1990). These observations strongly suggest that the standard practice of overspraying a plant with cold glyphosate at a field rate and then spotting the 14C-glyphosate on a particular leaf to measure translocation is a bad idea. First, the translocation from that source leaf will depend on āitsā perception of sink strengths based on its location on the plant. Second, the self-limitation due to whole plant toxicity will prematurely limit translocation. Third, the unknown proportional mixing of cold and 14C-glyphosate precludes learning about the concentration of glyphosate in a tissue. Because translocation studies are more concerned with how āmuchā glyphosate goes āwhereā from a source location, then one can simply apply a precise dose to a specific location. The faster the uptake, the better, because the first minute amounts of glyphosate delivered to sinks will begin to initiate the self-limitation, which ultimately stops translocation. Hence, a rapid delivery (but not locally cytotoxic) dose allows more glyphosate to be translocated and reveal the proportional sink strengths from that source location.
The use of GR plants compared with wild-type or a sensitive plant allows the separation of the effects of physiological barriers, like metabolic toxicity from physical barriers such as membranes, cell walls, and cuticles (Feng and Chiu 2005; Feng et al. 2003b). It is not always possible to have a GR plant for this comparison and so that situation can be created by using an ultralow dose of 14C-gly...
Table of contents
- Cover
- Half title page
- Title page
- Copyright page
- Dedication
- PREFACE
- ACKNOWLEDGMENTS
- CONTRIBUTORS
- 1 GLYPHOSATE: DISCOVERY, DEVELOPMENT, APPLICATIONS, AND PROPERTIES
- 2 HERBICIDE RESISTANCE: DEFINITIONS AND CONCEPTS
- 3 GLYPHOSATE-RESISTANT CROPS: DEVELOPING THE NEXT GENERATION PRODUCTS
- 4 TRANSITIONING FROM SINGLE TO MULTIPLE HERBICIDE-RESISTANT CROPS
- 5 TESTING METHODS FOR GLYPHOSATE RESISTANCE
- 6 BIOCHEMICAL MECHANISMS AND MOLECULAR BASIS OF EVOLVED GLYPHOSATE RESISTANCE IN WEED SPECIES
- 7 GLYPHOSATE RESISTANCE: GENETIC BASIS IN WEEDS
- 8 GENOMICS OF GLYPHOSATE RESISTANCE
- 9 GLYPHOSATE-RESISTANT CROP PRODUCTION SYSTEMS: IMPACT ON WEED SPECIES SHIFTS
- 10 GLYPHOSATE-RESISTANT HORSEWEED IN THE UNITED STATES
- 11 GLYPHOSATE-RESISTANT PALMER AMARANTH IN THE UNITED STATES
- 12 MANAGING GLYPHOSATE-RESISTANT WEEDS AND POPULATION SHIFTS IN MIDWESTERN U.S. CROPPING SYSTEMS
- 13 GLYPHOSATE-RESISTANT RIGID RYEGRASS IN AUSTRALIA
- 14 GLYPHOSATE RESISTANCE IN LATIN AMERICA
- 15 STRATEGIES FOR MANAGING GLYPHOSATE RESISTANCEā AN EXTENSION PERSPECTIVE
- 16 ECONOMIC IMPACT OF GLYPHOSATE-RESISTANT WEEDS
- Index