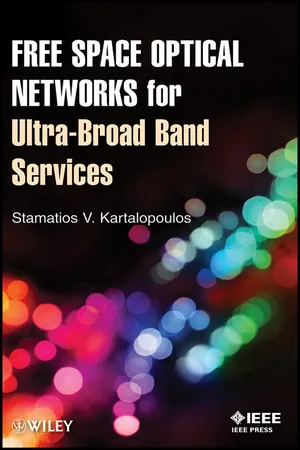
- English
- ePUB (mobile friendly)
- Available on iOS & Android
Free Space Optical Networks for Ultra-Broad Band Services
About This Book
This book provides a comprehensive description of an optical communications technology known as free space optical—a next-generation communications network that uses optical signals through the atmosphere instead of fiber, RF, or microwaves. This technology potentially offers more complex ultrabandwidth communication services simultaneously to multiple users and in a very short time, compared to fiber optic technology. This text presents established and new advancements drawn from the latest research and development in components, networking, operation, and practices.
This book describes the FSO network concepts in simple language. It provides comprehensive coverage in an easy-to-understand, progressive style that starts from the physics of the atmosphere and how it affects optical communications; continues with the design of a network node; and concludes with fiberless network applications from point-to-point to mesh topology. Important areas discussed include:
- Propagation of light in the atmosphere and phenomena that affect light propagation
- FSO transceiver design
- Point-to-point FSO systems
- Ring FSO systems
- Mesh-FSO systems and integrating the Mesh-FSO with the public network
- WDM Mesh-FSO
- FSO network security
- FSO-specific applications
To meet the needs of both academia and industry, key mathematical formulas are presented along with descriptions, while extensive mathematical analyses are minimized or avoided. Free Space Optical Networks for Ultra-Broad Band Services serves as an ideal text for network communication professionals who enter the free space optical communication field, graduate students majoring in optical communications, optical communication engineers, researchers, managers, and consultants.
Frequently asked questions
Information
- The 800 nm is generated by low cost vertical cavity surface emitting lasers (VCSEL) laser technology but the beam has low power and therefore the beam is modulated at very low data rates, up to 100 Mb/s and for link lengths of few hundred meters.
- The 1310 nm used to be a popular wavelength because of the distributed feedback (DFB) and Fabry-Perot type lasers, which support higher power than the VCSEL and therefore higher data rate and/or longer link lengths.
- The 1550 nm has been the most popular of all because it supports higher power levels, Gb/s data rate, longer link lengths, and also wavelength division multiplexing (WDM) technology [3, 4]; that is, several wavelengths in the 1520–1570 nm range and ITU-T standard compliant [5–8]; that is, an aggregate data rate which is the product of the number of different optical channels in the beam times the data rate in each channel. In some WDM applications, the 1310 nm is multiplexed with the 1550 nm to provide a two-channel WDM beam; this is acceptable in applications that do not require a large aggregate data rate; in addition, the 1310 and 1550 nm channels have large channel separation that turns out to be beneficial and convenient in receiver filter design. Moreover, a long-pass optical filter at the receiver rejects most wavelengths below the 1300 nm and it greatly reduces the solar background radiation (SBR) interference. The sun’s photosphere emits electromagnetic radiation in a wide spectrum that is centered at a wavelength of 500 nm (the visible spectrum is from 400 to 700 nm) and at an average temperature in excess of 5500°C; the sun’s radiation also includes wavelengths in the radio, Ultra Violet (UV), X-ray and Gamma-ray bands.







Table of contents
- Cover
- Series page
- Title page
- Copyright page
- Dedication
- PREFACE
- ACKNOWLEDGMENTS
- ABOUT THE AUTHOR
- INTRODUCTION
- 1 PROPAGATION OF LIGHT IN UNGUIDED MEDIA
- 2 FSO TRANSCEIVER DESIGN
- 3 POINT-TO-POINT FSO SYSTEMS
- 4 RING FSO SYSTEMS
- 5 MESH FSO SYSTEMS
- 6 WDM MESH-FSO
- 7 INTEGRATING MESH-FSO WITH THE PUBLIC NETWORK
- 8 FSO NETWORK SECURITY
- 9 FSO SPECIFIC APPLICATIONS
- ACRONYMS
- Index