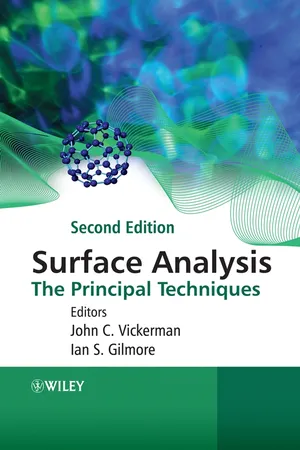
eBook - ePub
Surface Analysis
The Principal Techniques
This is a test
- English
- ePUB (mobile friendly)
- Available on iOS & Android
eBook - ePub
Surface Analysis
The Principal Techniques
Book details
Book preview
Table of contents
Citations
About This Book
This completely updated and revised second edition of Surface Analysis: The Principal Techniques, deals with the characterisation and understanding of the outer layers of substrates, how they react, look and function which are all of interest to surface scientists. Within this comprehensive text, experts in each analysis area introduce the theory and practice of the principal techniques that have shown themselves to be effective in both basic research and in applied surface analysis.
Examples of analysis are provided to facilitate the understanding of this topic and to show readers how they can overcome problems within this area of study.
Frequently asked questions
At the moment all of our mobile-responsive ePub books are available to download via the app. Most of our PDFs are also available to download and we're working on making the final remaining ones downloadable now. Learn more here.
Both plans give you full access to the library and all of Perlego’s features. The only differences are the price and subscription period: With the annual plan you’ll save around 30% compared to 12 months on the monthly plan.
We are an online textbook subscription service, where you can get access to an entire online library for less than the price of a single book per month. With over 1 million books across 1000+ topics, we’ve got you covered! Learn more here.
Look out for the read-aloud symbol on your next book to see if you can listen to it. The read-aloud tool reads text aloud for you, highlighting the text as it is being read. You can pause it, speed it up and slow it down. Learn more here.
Yes, you can access Surface Analysis by John C. Vickerman, Ian S. Gilmore, John C. Vickerman, Ian S. Gilmore in PDF and/or ePUB format, as well as other popular books in Technology & Engineering & Materials Science. We have over one million books available in our catalogue for you to explore.
Information
1
INTRODUCTION
The surface behaviour of materials is crucial to our lives. The obvious problems of corrosion are overcome by special surface treatments. The optical behaviour of glass can be modified by surface coatings or by changing the surface composition. The surface chemistry of polymers can be tuned so that they cling for packaging, are non-stick for cooking or can be implanted into our bodies to feed in drugs or replace body components. The auto-exhaust catalyst which removes some of the worst output of the combustion engine is a masterpiece of surface chemistry as are the industrial catalysts which are vital for about 90% of the output of the chemical industry. Thus whether one considers a car body shell, a biological cell, tissue or implant, a catalyst, a solid state electronic device or a moving component in an engine, it is the surface which interfaces with its environment. The surface reactivity will determine how well the material behaves in its intended function. It is therefore vital that the surface properties and behaviour of materials used in our modern world are thoroughly understood. Techniques are required which enable us to analyse the surface chemical and physical state and clearly distinguish it from that of the underlying solid.
1.1 How do we Define the Surface?
It is obvious that the surface properties of solids are influenced to a large extent by the solid state properties of the material. The question arises as to how we define the surface. Since the top layer of surface atoms are those that are the immediate interface with the other phases (gas, liquid or solid) impinging on it, this could be regarded as the surface. However, the structure and chemistry of that top layer of atoms or molecules will be significantly determined by the atoms or molecules immediately below. In a very real sense therefore, the surface could be said to be the top 2–10 atomic or molecular layers (say, 0.5–3 nm). However, many technologies apply surface films to devices and components, to protect, lubricate, change the surface optical properties, etc. These films are in the range 10–100 nm or sometimes even thicker, but the surface may be thought of in this depth range. However, beyond 100 nm it is more appropriate to begin to describe such a layer in terms of its bulk solid state properties. Thus we can consider the surface in terms of three regimes: the top surface monolayer, the first ten or so layers and the surface film, no greater than 100 nm. To understand fully the surface of a solid material, we need techniques that not only distinguish the surface from the bulk of the solid, but also ones that distinguish the properties of these three regimes.
1.2 How Many Atoms in a Surface?
It will be appreciated that it is not straightforward to probe a surface layer of atoms or molecules analytically and distinguish their structure and properties from that of the rest of the solid. One has only to consider the relatively small number of atoms involved in the surface layer(s) of an atomic solid to see that high sensitivity is required. How many atoms are we dealing with at the surface and in the bulk of a solid? We can consider a 1 cm cube of metal. One of the 1 cm2 surfaces has roughly 1015 atoms in the surface layer. Thus the total number of atoms in the cube will be ≈1023. Therefore the percentage of surface to bulk atoms will be:

Typically, a surface analysis technique may be able to probe in the region of 1mm2. Thus in the top monolayer there will be about 1013 atoms. In the top ten layers there will be 1014 atoms or 10−10 mol. Clearly in comparison with conventional chemical analysis we are considering very low concentrations. Things become more demanding when we remember that frequently the chemical species which play an important role in influencing surface reactivity may be present in very low concentration, so the requirement will be to analyse an additive or contaminant at the 10−3 or even 10−6 (ppm) atomic level, i.e. 1010 or 107 atoms or 10−14 or 10−17 mole levels respectively, perhaps even less.
Similar demands arise if the analysis has to be carried out with high spatial resolution. The requirement to map variations in chemistry across a surface can arise in a wide variety of technologies. There may be a need to monitor the homogeneity of an optical or a protective coating or the distribution of catalyst components across a support, a contaminant on an electronic device or a drug in a cell or tissue, etc. It is not unusual for 1 μm spatial resolution to be demanded, frequently even less would be beneficial. If we continue the discussion above in an area of 1 μm2 (10−12 m2 or 10−8 cm2) there are only ≈107 atoms, so if we want to analyse to the 10−3 atom fraction level, there are only 104 atoms. The nano particles that are part of many technologies these days present far fewer atoms for analysis, making the surface analysis task even more demanding.
Thus surface analysis is demanding in terms of its surface resolution and sensitivity requirements. However, there are in fact many surface analysis techniques, all characterized by distinguishing acronyms – LEED, XPS, AES, SIMS, STM, etc. Most were developed in the course of fundamental studies of surface phenomena on single crystal surface planes. Such studies which comprise the research field known as surface science seek to provide an understanding of surface processes at the atomic and molecular level. Thus for example, in the area of catalysis, there has been an enormous research effort directed towards understanding the role of surface atomic structure, composition, electronic state, etc. on the adsorption and surface reactivity of reactant molecules at the surface of the catalyst. To simplify and systematically control the variables involved, much of the research has focused on single crystal surfaces of catalytically important metals and more recently inorganic oxides. The surface analysis techniques developed in the course of these and related research are, in the main, based on bombarding the surface to be studied with electrons, photons or ions and detecting the emitted electrons, photons or ions.
1.3 Information Required
To understand the properties and reactivity of a surface, the following information is required: the physical topography, the chemical composition, the chemical structure, the atomic structure, the electronic state and a detailed description of bonding of molecules at the surface. No one technique can provide all these different pieces of information. A full investigation of a surface phenomenon will always require several techniques. To solve particular problems it is seldom necessary to have all these different aspects covered; however, it is almost always true that understanding is greatly advanced by applying more than one technique to a surface study. This book does not attempt to cover all the techniques in existence. A recent count identified over 50! The techniques introduced here are those (excluding electron microscopy which is not covered but for which there are numerous introductions) that have made the most significant impact in both fundamental and applied surface analysis. They are tabulated (via their acronyms) in Table 1.1 according to the principal information they provide and the probe/detection system they use. The number after each technique indicates the chapter in which it is described.
Table 1.1 Surface analysis techniques and the information they can provide
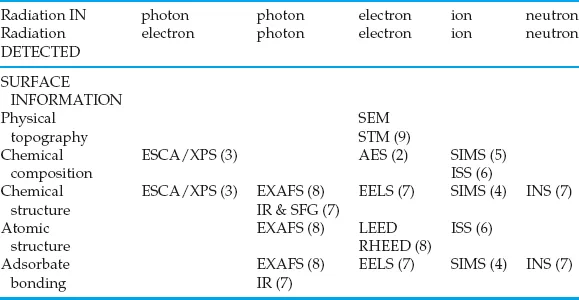
ESCA/XPS – Electron analysis for chemical analysis/X-ray photoelectron spectroscopy. X-ray photons of precisely defined energy bombard the surface, electrons are emitted from the orbitals of the component atoms, electron kinetic energies are measured and their electron binding energies can be determined enabling the component atoms to be determined.
AES – Auger electron spectroscopy. Basically very similar to the above except that a keV electron beam may be used to bombard the surface.
SIMS – Secondary ion mass spectrometry. There are two forms, i.e. dynamic and molecular SIMS. In both a beam of high energy (keV) primary ions bombard the surface while secondary atomic and cluster ions are emitted and analysed with a mass spectrometer.
ISS – Ion scattering spectrometry. An ion beam bombards the surface and is scattered from the atoms in the surface. The scattering angles and energies are measured and used to compute the composition and surface structure of the sample target.
IR – Infrared (spectroscopy). Various variants on the classical methods – irradiate with infrared photons which excite vibrational frequencies in the surface layers; photon energy losses are detected to generate spectra.
EELS – Electron energy loss spectroscopy. Low energy (few eV) electrons bombard the surface and excite vibrations – the resultant energy loss is detected and related to the vibrations excited.
INS – Inelastic neutron scattering. Bombard a surface with neutrons – energy loss occurs due to the excitation of vibrations. It is most efficient in bonds containing hydrogen.
SFG – Sum frequency generation. Two photons irradiate and interact with an interface (solid/gas or solid liquid) such that a single photon merges resulting in electronic or vibrational information about the interface region.
LEED – Low energy electron diffraction. A beam of low energy (tens of eV) electrons bombard a surface; the electrons are diffracted by the surface structure enabling the structure to be deduced.
RHEED – Reflection high energy electron diffraction. A high energy beam (keV) of electrons is directed at a surface at glancing incidence. The angles of electron scattering can be related to the surface atomic structure.
EXAFS – Extended X-ray absorption fine structure. The fine structure of the absorption spectrum resulting from X-ray irradiation of the sample is analysed to obtain information on local chemical and electronic structure.
STM – Scanning tunnelling microscopy. A sharp tip is scanned over a conducting surface at a very small distance above the surface. The electron current flowing between the surface and the tip is monitored; physical and electron density maps of the surface can be generated with high spatial resolution.
AFM – Atomic force microscopy (not included in table). Similar to STM but applicable to non-conducting surfaces. The forces developed between the surface and the tip are monitored. A topographical map of the surface is generated.
It is a characteristic of most techniques of surface analysis that they are carried out in vacuum. This is because electrons and ions are scattered by molecules in the gas phase. While photon based techniques can in principle operate in the ambient, sometimes gas phase absorption of photons can occur and as a consequence these may also require vacuum operation. This imposes a restriction on some of the surface processes that can be studied. For example, to study the surface gas or liquid interface it will usually be necessary to use a photon based technique, or one of the scanning probe techniques. Developments since the turn of the century are enabling the analysis of surfaces under ambient atmospheres using mass spectral methods analogous to SIMS (Chapter 4).
However, the vacuum based methods allow one to control the influence of the ambient on the surface under study. To analyse a surface uncontaminated by any adsorbate it is necessary to operate in ultra-high vacuum (<10−9 mmHg) since at 10−6 mmHg a surface can be covered by one mono-layer of adsorbed species within 1 s if the sticking coefficient (probability for adsorption) is 1. Controlled exposure of the surface to adsorbates or other surface treatments can then be carried out to monitor effects in a controlled manner. Appendix 1 on ‘Vacuum Technology’ will enable the reader to become familiar with the concepts and equipment requirements in the generation of vacua.
1.4 Surface Sensitivity
To generate the information, we require that a surface analysis technique should derive its data as near exclusively as possible from within the depth range discussed in Section 1.2. The extent to which a technique does this is a measure of its surface sensitivity. Ion scattering spectrometry (ISS) derives almost all its information from the top monolayer. It is very surface sensitive. Electron Spectroscopy for Chemical Analysis (ESCA) or X-ray Photoelectron Spectroscopy (XPS) samples the top ten or so layers of the surface, while infrared (IR) spectroscopy is not very surface sensitive and will sample deep into the solid, unless it is used as a reflection mode.
In general the surface sensitivity of an analytical method is dependent on the radiation detected. As already indicated, most of the methods of surface analysis involve bombarding the surface with a form of radiation – electrons, photons, ions, neutrons – and then collecting the resulting emitted radiation – electrons, photons, ions, neutrons. The scanning probe methods are a little different, although one could say that scanning tunnelling microscopy (STM) detects electrons. (Atomic force microscopy monitors the forces between the surface and a sharp tip, see Chapter 9.) The surface sensitivity depends on the depth of origin of the detected species. Thus in XPS while the X-ray photons which bombard the surface can penetrate deep into the solid, the resultant emitted electrons which can be detected without loss of energy can only arise from within 1–4 or 8 nm of the surface. Electrons generated deeper in the solid may escape, but on the way out they will have collided with other atoms and lost energy. They are no use for analysis. Thus the surface sensitivity of ESCA is a consequence of the short distance electrons can travel in solids without being scattered (known as the inelastic mean free path). Similarly, in secondary ion mass spectrometry (SIMS) the surface is bombarded by high energy ions. They deposit their energy down to 30 or 40 nm. However, 95% of the secondary ions that are knocked out (sputtered) of the solid arise from the top two layers.
There are techniques like infrared (IR) spectroscopy which, although they are not intrinsically very surface sensitive, can be made so by the methods used to apply them. Thus with IR a reflection approach can be used in which the incoming radiation is brought in at a glancing incidence. This enables vibrational spectra to be generated from adsorbates on single crystal surfaces. The technique is very surface sensitive. Surface sensitivity can be significantly increased even in surface sensitive methods like ESCA by irradiating the surface at glancing incidence – see Chapter 3.
Various terms are used to define surface sensitivity. With all the techniques described in this book the total signal detected will originate over a range of depths from the surface. An information depth may be specified which is usually defined as the average distance (in nm) normal to the surface from which a specified percentage (frequently 90, 95 or 99%) of the detected signal originates. Sometimes, as in ESCA, a sampling depth, is defined. This is three times the inelastic mean free path, and turns out to be the information depth where the percentage is 95%. Obviously a very small proportion of the detected signal does arise from deeper in the solid, but the vast majority of the useful analytical information arises from within the sampling depth region.
In molecular SIMS the information depth is the depth from which 95% of the secondary ions originate. For most materials this is believed to be about two atomic layers, about 0.6 nm. However, it is sometimes difficult to be sure what a layer is. For example, there are surface layers used to generate new optical properties that are composed of long organic chains bonded to metal or oxide surfaces. The organic layer is much less dense than the substrate underneath. SIMS studies of these materials suggest that the analytical process may remove the whole molecular chain which can easily be >20 nm long. Surface sensitivity in this case is a very different concept from that which would apply to the surface of a metal or inorganic compound.
1.5 Radiation Effects – Surface Damage
To obtain the surface information required entails ‘interfering’ with the surface state in some way! Most of the techniques require the surface to be bombarded with photons, electrons or ions. They will affect the chemical and physical state of the surface being analysed. Thus in the course of analysing the surface, the surface may be changed. It is important to understand the extent to which this may happen, otherwise the information being generated from the surface may not be characteristic of the surface before analysis; rather it may reflect a surface damaged by the incident radiation.
Table 1.2 shows the penetration depth and influence of the 1000 eV particles. It can be seen that most of the energy is deposited in the near surface under ion and electron bombardment, so in general terms it would be expected that the extent of surface damage would vary as photons < electrons < ions. Consequently, it is sometimes carelessly suggested that ESCA/XPS is a low damage technique. However, the power input to the surface in the course of an experiment is considerably less in the ion bombardment method of SIMS compared to photon bombardment in ESCA (Table 1.3). SIMS is very obviously a phenomenon that depends on damage – ions bombard to knock out other ions! Without damage there is no information, but as will be seen in Chapter 4 it can be operated in a low damage mode to generate significant surface information. The X-ray photons which bombard the surface in XPS penetrat...
Table of contents
- COVER
- TITLE
- COPYRIGHT
- CONTRIBUTORS
- PREFACE
- 1: INTRODUCTION
- 2: AUGER ELECTRON SPECTROSCOPY
- 3: ELECTRON SPECTROSCOPY FOR CHEMICAL ANALYSIS
- 4: MOLECULAR SURFACE MASS SPECTROMETRY BY SIMS
- 5: DYNAMIC SIMS
- 6: LOW-ENERGY ION SCATTERING AND RUTHERFORD BACKSCATTERING
- 7: VIBRATIONAL SPECTROSCOPY FROM SURFACES
- 8: SURFACE STRUCTURE DETERMINATION BY INTERFERENCE TECHNIQUES
- 9: SCANNING PROBE MICROSCOPY
- 10: THE APPLICATION OF MULTIVARIATE DATA ANALYSIS TECHNIQUES IN SURFACE ANALYSIS
- APPENDIX 1: VACUUM TECHNOLOGY FOR APPLIED SURFACE SCIENCE
- APPENDIX 2: UNITS, FUNDAMENTAL PHYSICAL CONSTANTS AND CONVERSIONS
- INDEX