
eBook - ePub
Power Ultrasound in Electrochemistry
From Versatile Laboratory Tool to Engineering Solution
This is a test
- English
- ePUB (mobile friendly)
- Available on iOS & Android
eBook - ePub
Power Ultrasound in Electrochemistry
From Versatile Laboratory Tool to Engineering Solution
Book details
Book preview
Table of contents
Citations
About This Book
The use of power ultrasound to promote industrial electrochemical processes, or sonoelectrochemistry, was first discovered over 70 years ago, but recently there has been a revived interest in this field. Sonoelectrochemistry is a technology that is safe, cost-effective, environmentally friendly and energy efficient compared to other conventional methods.
The book contains chapters on the following topics, contributed from leading researchers in academia and industry:
- Use of electrochemistry as a tool to investigate Cavitation Bubble Dynamics
- Sonoelectroanalysis
- Sonoelectrochemistry in environmental applications
- Organic Sonoelectrosynthesis
- Sonoelectrodeposition
- Influence of ultrasound on corrosion kinetics and its application to corrosion tests
- Sonoelectropolymerisation
- Sonoelectrochemical production of nanomaterials
- Sonochemistry and Sonoelectrochemistry in hydrogen and fuel cell technologies
Frequently asked questions
At the moment all of our mobile-responsive ePub books are available to download via the app. Most of our PDFs are also available to download and we're working on making the final remaining ones downloadable now. Learn more here.
Both plans give you full access to the library and all of Perlegoâs features. The only differences are the price and subscription period: With the annual plan youâll save around 30% compared to 12 months on the monthly plan.
We are an online textbook subscription service, where you can get access to an entire online library for less than the price of a single book per month. With over 1 million books across 1000+ topics, weâve got you covered! Learn more here.
Look out for the read-aloud symbol on your next book to see if you can listen to it. The read-aloud tool reads text aloud for you, highlighting the text as it is being read. You can pause it, speed it up and slow it down. Learn more here.
Yes, you can access Power Ultrasound in Electrochemistry by Bruno Pollet in PDF and/or ePUB format, as well as other popular books in Physical Sciences & Physical & Theoretical Chemistry. We have over one million books available in our catalogue for you to explore.
Information
1
An Introduction to Sonoelectrochemistry
1.1 Introduction to Ultrasound and Sonochemistry
Ultrasound is defined as sound with a frequency that is beyond the range of human hearing and this is generally considered to be above 20 kHz (20 000 hertz, hertz = cycle per second). The ultrasound frequency range can be broadly subdivided into two categories (Figure 1.1):
i. Diagnostic ultrasound operates at very high frequencies (above 5 MHz); it is used in foetal scanning and in general for the non-destructive testing of materials. The important feature of diagnostic ultrasound is that the energy involved is too low to produce cavitation in tissues and so is safe to use in medical scanning.
Figure 1.1 Sound range.
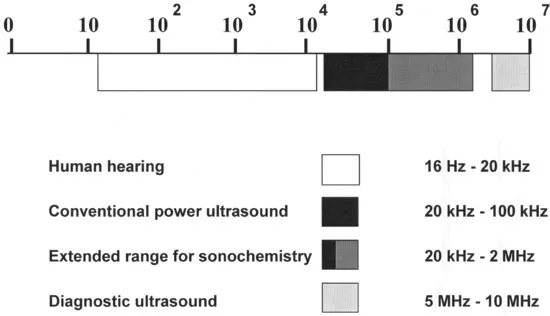
ii. Power ultrasound is mainly in the frequency range between 20 and 100 kHz (but can be extended up to 2 MHz). The energies involved are sufficiently high to produce cavitation in liquids and thus can be used to influence chemistry and processing (see later).
The origins of ultrasound can be traced back to the late nineteenth century with the discovery of the piezoelectric effect by Curie and the ultrasonic whistle by Galton in 1893. However, the first commercial application of ultrasonics did not appear until 1917 with Langevin's echo sounding technique. Langevin's discovery was the direct result of a competition organised in 1912 to find a method of detecting icebergs and so to avoid any repetition of the disaster which befell the Titanic. Essentially all imaging, from medical ultrasound to non-destructive testing (flaw detection), relies upon the same pulse echo type of approach, that is, a pulse of sound is transmitted through a material and is reflected from a boundary. The time of flight from emission to reception of the echo is then used to compute the distance to the boundary using the speed of sound in that medium. High frequency ultrasound (>1 MHz) is therefore employed for modern medical imaging and non-destructive testing.
The potential of using acoustic cavitation to create changes in materials was identified in the USA in the late 1920s [1â3]. Over the succeeding years some more research went into the applications of ultrasound in chemistry and the first review of this type of work was published in the 1940s [4].
By the 1950s, industry had adopted power ultrasound (which at that time was known as âMacrosonicsâ) for a range of applications that were gathered together in a series of five publications [5]. At that time there were six general industrial applications and these are listed in order of commercial turnover in Table 1.1.
Table 1.1 Industrial applications of macrosonics: the major established areas. Reprinted with permission from Ultrasonics, Macrosonics in Industry 1. Introduction by E. A. Neppiras, 10, 1, 9â13 Copyright (1972) Elsevier Ltd.
1 | Cleaning | Dispersing loosely held non-soluble particles and coatings Dissolving solid surface coatings Degreasing Descaling |
2 | Plastic Welding | Remote welding of rigid thermo-plastics Insertion Staking Splicing Continuous welding of sheet material |
3 | Metal Welding | Microbonding Continuous welding of sheet material Bonding metals to non-metals |
4 | Chemical Processing | Extracting: â perfume from flowers essential oils from hops juices from fruits chemicals from plants biomacromolecules from cells |
5 | Metal Forming | Wire-drawing Tube-drawing Plastic-forming of sheet metal |
6 | Machining | Ultrasonic machining with abrasive Vibration-assisted ECM Vibration-assisted milling, turning, drilling, grinding, polishing Teeth descaling Vibration-assisted rotary drills |
The majority of the applications shown in Table 1.1 can be considered to be âengineeringâ and the whole area of ultrasonic applications in the chemistry laboratory, which had been identified in the 1940s, did not really develop until the 1980s. This renaissance is generally considered to be due to chemists becoming aware of potential alternative uses of ultrasonic equipment which was then appearing in the laboratory in the form of cleaning baths and ultrasonic probes (the latter more commonly in biological laboratories in the form of biological cell disruptors).
From Table 1.1, it can be seen that power ultrasound can be used in two basic ways to bring about changes in a material and these are:
i. Direct mechanical transmission of vibration from the transducer onto a solid to induce vibrations in the surface of the material.
ii. Indirect transmission of energy via cavitation induced in a fluid by the transmission of acoustic vibrations.
Each of these has a relevance to electrochemistry since (i) can be taken to represent induced vibrations at a solid electrode surface and (ii) can be taken to represent sonication of an electrolyte. In many cases both will occur simultaneously and influence the overall electrochemical process.
1.2 Applications of Power Ultrasound through Direct Vibrations
The application of power ultrasound through the introduction of direct vibrations onto a solid surface is the basis of many engineering applications including welding, drilling, cutting and tube drawing. The drilling and cutting applications have now also become an important development in surgery.
1.2.1 Welding
A large proportion of ultrasonic equipment currently in industry is devoted to welding or riveting plastic mouldings for the consumer market. The equipment generally operates at around 20 kHz and a shaped tool or horn transmits (and amplifies) the vibrating motion to a shaped die pressing together the two pieces of material to be welded. The vibrational amplitude is typically 50â100 microns (Îźm).
Ultrasonic welding is generally used for the more rigid amorphous types of thermoplastic. It is particularly important that the vibrational energy is primarily transmitted to the joint rather than be absorbed by the body of the material, producing heat. This is because any warming of the bulk material can lead to a release of internal moulding stresses and produce distortion. Thermoplastics have two properties which make them particularly suited to ultrasonic welding: (a) low thermal conductivity and (b) melting or softening temperatures of between 100 and 200 °C. As soon as the ultrasonic power is switched off the substrate or bulk material becomes a heat-sink, giving rapid cooling of the welded joint. When the more traditional conductive heating is used for welding, however, the thermal gradient has to be reversed before cooling occurs, leading to long heating/cooling process cycles. Another major advantage of the use of ultrasound is the high joint strength of the weld, reaching 90â98% of the material strength. Indeed, test samples usually break in the body of the material and not at the weld itself.
1.2.1.1 Machining (Drilling and Cutting)
Ultrasonic drilling and cutting were originally introduced and developed for the accurate profiling of brittle materials such as ceramics and glass. It has been used in the aerospace industry since the 1970s for cutting glass and carbon fibre composites and has found many applications in the food industry. In dentistry it is used for removing plaque from teeth and in medicine it is used to improve the efficiency of scalpels and for bone cutting.
Ultrasonic cutting uses a knife-type blade attached through a shaft to an ultrasonic source. Essentially the shaft with its blade behaves as an ultrasonic horn and is normally operated at a frequency of 20 kHz. The cutting action is a combination of the pressure applied to the sharp cutting edge surface and the mechanical longitudinal vibration of the blade. Typically the tip movement will be in the range 50 to 100 microns peak to peak. Several advantages arise from this technology:
i. The ultrasonic vibration of 20 kHz applies an intermittent for...
Table of contents
- Cover
- Title Page
- Copyright
- Dedication
- Foreword
- About the Editor
- List of Contributors
- Acknowledgements
- Introduction to Electrochemistry
- 1: An Introduction to Sonoelectrochemistry
- 2: The Use of Electrochemistry as a Tool to Investigate Cavitation Bubble Dynamics
- 3: Sonoelectroanalysis: An Overview
- 4: Sonoelectrochemistry in Environmental Applications
- 5: Organic Sonoelectrosynthesis
- 6: Sonoelectrodeposition: The Use of Ultrasound in Metallic Coatings Deposition
- 7: Influence of Ultrasound on Corrosion Kinetics and its Application to Corrosion Tests
- 8: Sonoelectropolymerisation
- 9: Sonoelectrochemical Production of Nanomaterials
- 10: Sonochemistry and Sonoelectrochemistry in Hydrogen and Fuel Cell Technologies
- Appendix: Sonochemical Effects on Electrode Kinetics
- Index