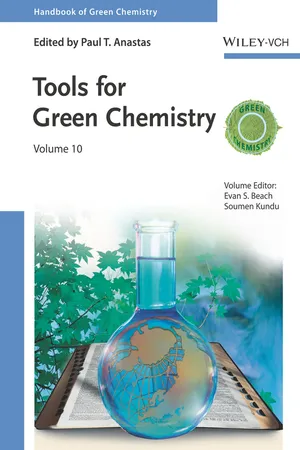
This is a test
- English
- ePUB (mobile friendly)
- Available on iOS & Android
eBook - ePub
Tools for Green Chemistry, Volume 10
Book details
Book preview
Table of contents
Citations
About This Book
Volume 10 in the Handbook of Green Chemistry series provides useful and practical tools, databases, and laboratory approaches to support chemists working in both academia and industry in achieving their green chemistry goals. Among many other helpful techniques covered, the authors offer prediction software, life cycle assessment methodology, and screening tools.
Frequently asked questions
At the moment all of our mobile-responsive ePub books are available to download via the app. Most of our PDFs are also available to download and we're working on making the final remaining ones downloadable now. Learn more here.
Both plans give you full access to the library and all of Perlegoâs features. The only differences are the price and subscription period: With the annual plan youâll save around 30% compared to 12 months on the monthly plan.
We are an online textbook subscription service, where you can get access to an entire online library for less than the price of a single book per month. With over 1 million books across 1000+ topics, weâve got you covered! Learn more here.
Look out for the read-aloud symbol on your next book to see if you can listen to it. The read-aloud tool reads text aloud for you, highlighting the text as it is being read. You can pause it, speed it up and slow it down. Learn more here.
Yes, you can access Tools for Green Chemistry, Volume 10 by Evan S. Beach, Soumen Kundu, Paul T. Anastas in PDF and/or ePUB format, as well as other popular books in Physical Sciences & Environmental Science. We have over one million books available in our catalogue for you to explore.
Information
1
Application of Life Cycle Assessment to Green Chemistry Objectives
Thomas E. Swarr, Daniele Cespi, James Fava, and Philip Nuss
1.1 Introduction
Green chemistry (GC) is described by the 12 principles of green chemistry to guide the design of chemical products and processes that reduce or eliminate the generation and use of hazardous substances [1]. The guiding principles have been criticized for being qualitative and failing to provide an objective means to assess the overall âgreennessâ of proposed solutions or to evaluate trade-offs among conflicting principles, for example, reduced toxicity, but increased energy consumption [2]. Life cycle assessment (LCA) is the âcompilation and evaluation of the inputs, outputs, and the potential environmental impacts of a product systemâ and provides a quantitative method to address these concerns (3], p.2). It is an international standard recognized as an effective methodology to evaluate improvement strategies and avoid shifting problems to other times and places or among various environmental media [4,5].
LCA, however, has its own set of limitations and unresolved methodology issues [6,7]. Some are particularly relevant to green chemistry, such as limited data for chemical production chains, lack of geographic specificity, and aggregation of emissions over time [8â10]. Increasingly, researchers are recognizing that the strengths and weaknesses of GC and LCA are complementary and are advocating for more effective integration of both methodologies to develop more sustainable solutions [2,11,12]. Anastas and Lankey (11], p.289) broaden the definition of green chemistry by considering chemistry to include âthe structure and transformation of all matterâ and hazardous impacts to address the âfull range of threats to human health and the environment.â Application of LCA to GC problems promises a better understanding of the flow of toxics through the economy and provides a robust framework for organizing knowledge about inherent hazards associated with product systems [13].
LCA is comprised of four basic steps [3]. Goal and scope identifies the purpose of the study, how the results will be used, and intended audience to whom the results will be communicated. Clear definition of the decision context is critical to ensure the study provides objective information that enables the study commissioner and intended audience to make informed choices according to their values and priorities. Life cycle inventory (LCI) gathers data necessary to model mass and energy flows across the entire product system, from extraction or harvest of resources to the ultimate disposal of the product. Realistically, these models are always incomplete and a key decision is where to draw the boundaries on what is included in the system model. Life cycle impact assessment (LCIA) evaluates the significance of exchanges between the product system and the natural environment. Environmental interchanges are grouped, or classified into impact categories, such as acidification, climate change, human toxicity, or resource depletion. The inventory items for each impact category are then characterized for potency and mass, often in terms of a reference substance. For example, different greenhouse gases have different warming potentials, and are converted to an equivalent mass of CO2, allowing the aggregated emission to be expressed as CO2(e) (equivalents). Finally, interpretation attempts to make sense of the analytical results to provide conclusions and recommendations necessary to satisfy the intended goal and scope of the study. For additional background on LCA methods relevant to GC, the reader is referred to previous reviews. Kralisch et al. [12] provides a general overview of LCA, specific considerations to be considered in chemical design, and examples of applications to emerging research problems. Tufvesson et al. [9] reviews âgreen chemistryâ LCA studies to identify key parameters and methodological issues.
Principles of Green Chemistry
- Prevention
- Atom economy
- Less hazardous chemical syntheses
- Designing safer chemicals
- Safer solvents and auxiliaries
- Design for energy efficiency
- Use of renewable feedstocks
- Reduce derivatives
- Catalysis
- Design for degradation
- Real-time analysis for pollution prevention
- Inherently safer chemistry for accident prevention
The present chapter is intended to provide guidance on the effective integration of LCA methods into GC initiatives. It is assumed that the primary audience is a practitioner in green chemistry, familiar with the 12 principles of green chemistry, and perhaps with only a basic understanding of LCA methods. (Because the principles are sometimes listed differently, the American Chemistry Society version is reproduced in the text box.)1 There is no attempt to provide a comprehensive review of current state of the art in green chemistry progress or of the latest developments in LCA. Illustrative case studies or research results are presented to emphasize key points in the application of LCA methods to GC objectives. The chapter is organized based on grouping GC objectives into the following three overarching categories:
- Substitution of hazardous chemicals with safer alternatives (Principles 3,4,5,10,11,12).
- Design of processes to be more energy and material efficient (Principles 1,2,6,8,9,11).
- Promoting a transition to renewables (materials and energy) (Principles 6,7).
Further, Sjöström [14] characterized GC as a meta-discipline and described a classification model of GC research, management, and policy activities. Thus, the applicability of LCA to these various activities is also addressed for the various GC objectives.
Section 1.2 on substitution addresses the challenge of quantifying the toxicity of chemicals and the products and processes that depend on those chemicals. There are fundamental differences between LCA and GC or chemical alternative assessments. While these are typically focused on use of a chemical to provide a required function in a specific application, LCA considers use of the chemical (along with other chemicals) in a product system designed to satisfy some end user need or function. Thus, the two methodologies are designed to answer different questions, and this must be considered in the application of LCA to quantify toxicity concerns. Section 1.3 on greener processes builds on this introduction to consider a broader range of environmental impacts to assess trade-offs among the various GC principles and evaluate the overall greenness of a product or process. LCA provides a robust method to quantitatively compare alternative solutions, but does not provide guidance on development of alternatives. GC complements LCA by providing specific guidance to address the issues identified in the study. Section 1.4 addresses the broad goal of promoting renewable materials and energy. This class of problems extends system considerations to include a wide range of environmental impacts and dynamic effects, such as indirect land use changes that go well beyond traditional GC assessments. Given the importance of climate change concerns, determining whether renewables provide a real benefit over synthetic alternatives is a critical area for future work. Finally, Section 1.5 concludes with recommendations to promote the effective integration of LCA and GC to develop more sustainable business practices.
1.2 Substitution of Safer Chemicals
A variety of chemical alternative assessment procedures have been developed to guide selection of safer substitutes [15â17]. These methods typically begin with identifying a target chemical of concern followed by an analysis of the uses of the chemical. Virtually all methods advocate âlife cycle thinking,â but the focus on a specific chemical can narrow the problem definition. Alternatives are identified based on satisfying the same technical requirements for the application, or use under consideration. LCA, by contrast, has traditionally been focused on product systems and the function or service provided to the customer or end user. This broader perspective can inspire innovative approaches to satisfy the end user demand with an alternative solution that does not rely on the chemical of concern, thus eliminating the need for an alternatives assessment. If the assessment cannot identify an alternative that satisfies the technical requirements, then the analyst is advised to implement best practices to limit human exposures and environmental releases and to continue to research alternatives.
The use of comprehensive LCA studies in early development stages is often dismissed as being overly complex and time and effort intensive [12]. Problems gathering inventory data for chemicals, and particularly for fine chemicals have been well documented [9,18]. Inventory data is often protected as proprietary business information. Fine chemicals tend to be produced in smaller batches, comprised of many processing steps, and produced in shared equipment in multiproduct facilities, with much data collected only at the facility level. A case study comparing alternative assessment tools for the characterization of organic solvents concluded use of LCA was limited due to data constraints that included both inventory data for the production of chemicals and characterization factors for toxicity of chemicals [19].
1.2.1 Missing Inventory Data and Characterization Factors
Researchers have developed methods to fill inventory data gaps in chemical production systems using basic knowledge of chemical processes and proxy data base...
Table of contents
- Cover
- Related Titles
- Title Page
- Copyright
- About the Editors
- List of Contributors
- Preface
- Chapter 1: Application of Life Cycle Assessment to Green Chemistry Objectives
- Chapter 2: Shortcut Models Based on Molecular Structure for Life Cycle Impact Assessment: The Case of the FineChem Tool and Beyond
- Chapter 3: Models to Estimate Fate, Exposure, and Effects of Chemicals
- Chapter 4: Collaborative Approaches to Advance Chemical Safety
- Chapter 5: Introduction to Green Analytical Chemistry
- Chapter 6: Cosmo-RS-Assisted Solvent Screening for Green Extraction of Natural Products
- Chapter 7: Supramolecular Catalysis as a Tool for Green Chemistry
- Chapter 8: A Tutorial of the Inverse Molecular Design Theory in Tight-Binding Frameworks and Its Applications
- Chapter 9: Green Chemistry Molecular Recognition Processes Applied to Metal Separations in Ore Beneficiation, Element Recycling, Metal Remediation, and Elemental Analysis
- Chapter 10: Shaping the Future of Additive Manufacturing: Twelve Themes from Bio-Inspired Design and Green Chemistry
- Chapter 11: The IFF Green Chemistry Assessment Tool
- Index
- End User License Agreement