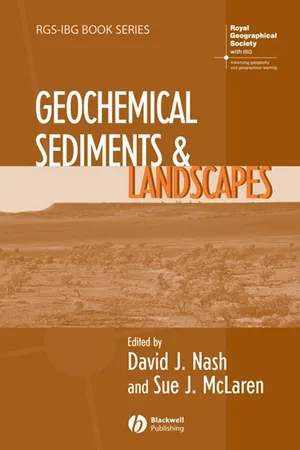
This is a test
- English
- ePUB (mobile friendly)
- Available on iOS & Android
eBook - ePub
Geochemical Sediments and Landscapes
Book details
Book preview
Table of contents
Citations
About This Book
This state-of-the-art volume reviews both past work and current research, with contributions from internationally recognized experts. The book is organized into fourteen chapters and designed to embrace the full range of terrestrial geochemical sediments.
- An up-to-date and comprehensive survey of research in the field of geochemical sediments and landscapes
- Discusses the main duricrusts, including calcrete, laterite and silcrete
- Considers deposits precipitated in various springs, lakes, caves and near-coastal environments
- Considers the range of techniques used in the analysis of geochemical sediments, representing a significant advance on previous texts
Frequently asked questions
At the moment all of our mobile-responsive ePub books are available to download via the app. Most of our PDFs are also available to download and we're working on making the final remaining ones downloadable now. Learn more here.
Both plans give you full access to the library and all of Perlegoâs features. The only differences are the price and subscription period: With the annual plan youâll save around 30% compared to 12 months on the monthly plan.
We are an online textbook subscription service, where you can get access to an entire online library for less than the price of a single book per month. With over 1 million books across 1000+ topics, weâve got you covered! Learn more here.
Look out for the read-aloud symbol on your next book to see if you can listen to it. The read-aloud tool reads text aloud for you, highlighting the text as it is being read. You can pause it, speed it up and slow it down. Learn more here.
Yes, you can access Geochemical Sediments and Landscapes by David J. Nash, Sue J. McLaren in PDF and/or ePUB format, as well as other popular books in Physical Sciences & Geology & Earth Sciences. We have over one million books available in our catalogue for you to explore.
Information
Chapter One
Introduction: Geochemical Sediments in Landscapes
1.1 Scope of This Volume
Geochemical sediments of various types are an often overlooked but extremely important component of global terrestrial environments. Where present, chemical sediments and residual deposits may control slope development and landscape evolution, increase the preservation potential of otherwise fragile sediments, provide important archives of environmental change, act as relative or absolute dating tools and, in some cases, be of considerable economic importance. Chemical sedimentation may occur in almost any terrestrial environment, providing there is a suitable dissolved mineral source, a mechanism to transfer the mineral in solution to a site of accumulation and some means of triggering precipitation. However, given the increased importance of chemical weathering in the tropics and sub-tropics, they tend to be most widespread in low-latitude regions (Goudie, 1973).
Despite their global significance, terrestrial geochemical sediments have not been considered collectively for over 20 years. Indeed, the last book to review the full suite of chemical sediments and residual deposits was Goudie and Pyeâs seminal volume Chemical Sediments and Geomorphology (Goudie and Pye, 1983a). Since then, selected geochemical sediments have been discussed in volumes such as Wright and Tucker (Calcretes; 1991), Martini and Chesworth (Weathering, Soils and Palaeosols; 1992), Ollier and Pain (Regolith, Soils and Landforms; 1996), Thiry and Simon-Coinçon (Palaeoweathering, Palaeosurfaces and Related Continental Deposits; 1999), Dorn (Rock Coatings; 1998), Taylor and Eggleton (Regolith Geology and Geomorphology; 2001), and Chen and Roach (Calcrete: Characteristics, Distribution and Use in Mineral Exploration; 2005). However, many of these texts tend to discuss geochemical sediments within either a geological or pedological framework, often with little attempt to position them in their geomorphological context. As will be seen in section 1.3 and many of the chapters in this volume, understanding the influence of landscape setting upon geochemical sedimentation is of paramount importance if the resulting chemical sediments and residua are to be correctly interpreted. The need for a follow-up volume to Goudie and Pye (1983a) became very apparent during meetings of the British Geomorphological Research Group (BGRG) fixed-term working group on Terrestrial Geochemical Sediments and Geomorphology, convened by the editors and Andrew Goudie, which ran between 2001 and 2004. Indeed, the majority of the authors within this collection were members of the working group, and all royalties from this book will go to the BGRG (now the British Society for Geomorphology).
The individual chapters within Geochemical Sediments and Landscapes focus largely on the relationships between geomorphology and geochemical sedimentation. Given the emphasis on landscape, the range of precipitates and residual deposits considered are mainly those which form in terrestrial settings. An exception is the chapter on beachrock and intertidal precipitates (Gischler, Chapter 11), which develop at the terrestrial-marine interface but, where present, have a significant impact upon coastal geomorphology and sedimentology. The definition of geochemical sediments used in the volume is a deliberately broad one, reflecting the wide range of environments under which chemical sedimentation can occur. As Goudie and Pye (1983b) suggest, geochemical sediments are conventionally defined as sedimentary deposits originating through inorganic chemical processes. This distinguishes them from clastic, volcaniclastic, biochemical and organic sediments. However, this definition is not especially useful, since the majority of the geochemical sediments reviewed here comprise a mixture of detrital clastic particles which are bound together by various intergranular chemical precipitates. Certainly, there are some very âpureâ chemical precipitates, such as speleothems (see Fairchild et al., Chapter 7) and some lacustrine deposits (Verrecchia, Chapter 9), but these are the exception rather than the rule. The conventional definition also places greatest emphasis on the role of physico-chemical processes in geochemical sedimentation. However, as will be seen from many chapters in this collection, biogeochemical processes are increasingly recognised as being of vital importance for the formation of a wide range of supposedly âchemicalâ precipitates. Indeed, biological agencies may be directly implicated in the formation of many chemical sediments, and play a key role in the weathering and release of solutes for a wide range of other precipitates.
1.2 Organisation
Geochemical Sediments and Landscapes is organised into 14 chapters. These are arranged so that the main duricrusts (calcrete, laterite and silcrete) are discussed first (Chapters 2â4), followed by a consideration of deposits precipitated in various aeolian, slope, spring, fluvial, lake, cave and nearcoastal environments (Chapters 5â12). The volume concludes with an overview of the range of techniques available for analysing geochemical sediments (McAlister and Smith, Chapter 13) and a general summary which includes a consideration of directions for future research (McLaren and Nash, Chapter 14).
The specific content of individual chapters, inevitably, reflects the primary research interests of the contributing authors. However, all contributors were requested, where appropriate, to include information about the nature and general characteristics, distribution, field occurrence, landscape relations, macro- and micromorphology, chemistry, mineralogy, mechanisms of formation or accumulation, and palaeoenvironmental significance of their respective geochemical sediment. Individual deposits are treated as discrete entities in their specific chapters. However, in recognition of the fact that individual chemical sediments may grade laterally or vertically into geochemically allied materials, for example along pH (e.g. calcrete and silcrete) or other environmental gradients (e.g. beachrock and coastal aeolianite), authors were also asked to highlight any significant relationships to other terrestrial geochemical sediments. Despite its title, the chapters within Geochemical Sediments and Landscapes do not include lengthy discussions of the physics of geochemical sedimentation; authors were instead asked to cite suitable references so that interested readers can access such materials.
1.3 Significance of Geochemical Sediments in Landscapes
The geochemical precipitates and residual deposits discussed within this volume are significant from a range of geomorphological, palaeoenvironmental and economic perspectives. From a geomorphological standpoint, the more indurated and resistant chemical sediments such as calcrete (Wright, Chapter 2), ferricrete (Widdowson, Chapter 3) and silcrete (Nash and Ullyott, Chapter 4) exert a major influence upon the topographic evolution of many parts of the world. This influence is most noticeable in tropical and sub-tropical areas because such duricrusts are most widespread in these regions (Goudie, 1973). Geochemical crusts that have developed over palaeosurfaces may be preserved as horizontal to sub-horizontal caprocks on plateaux and mesas (Goudie, 1984). Along the southern coast of South Africa, for example, silcrete and ferricrete accumulation within deeply weathered bedrock has led to the preservation of remnants of the post-Gondwana âAfrican Surfaceâ (Summerfield, 1982, 1983a; Marker et al., 2002). In contrast, where geochemical sediment formation took place preferentially in a topographic low, usually as a product of groundwater-related cementation, relief and drainage inversion may occur if surrounding uncemented and less resistant materials are removed by erosion (Pain and Ollier, 1995). In Australia, silcretes developed within palaeochannels may now crop out in inverted relief (e.g. Barnes and Pitt, 1976; Alley et al., 1999; Hill et al., 2003). In either case, the presence of a duricrust caprock exerts a control upon slope development and hydrology and may significantly retard landscape denudation. The undercutting and subsequent collapse of caprocks may lead to the development of characteristic features such as âbreakawaysâ with the resulting slope surfaces mantled by duricrust-derived regolith.
Geochemical sedimentation may also play a more subtle but equally important role in preserving âephemeralâ sediment bodies which would otherwise be highly susceptible to erosion and destruction. Calcium carbonate, gypsum or halite cementation of near-coastal and desert dune sands may, for example, significantly enhance their preservation potential once they are transformed to aeolianite (e.g. McKee, 1966; Gardner, 1998; McLaren and Gardner, 2004; see McLaren, Chapter 5). Similarly, the induration of fluvial terrace sediments through the development of pedogenic or groundwater calcretes may increase their resistance to erosion and reworking and hence preserve key palaeohydrological evidence (e.g. Candy et al., 2004a; see Wright, Chapter 2). In extreme cases, geochemical sedimentation may lead to the complete preservation of relict landforms, as, for example, in the case of the silica- and carbonate cemented palaeochannels described by Maizels (1987, 1990) from central Oman.
In addition to their geomorphological roles, chemical sediments of various types may act as important archives of palaeoenvironmental information. Even in the most arid deserts, where detailed hydrological or climatic data are often sparse, the occurrence of crusts such as calcrete or gypcrete at or near the land surface is a clear indication that the mobilisation and precipitation of minerals in the presence of water has occurred in the past. Evaporites in Death Valley, USA, for example, have been used to unravel sequences of regional climatic changes over the past 200,000 years (Lowenstein et al., 1999; see Chivas, Chapter 10). The accumulation of thick sequences of geochemical precipitates usually requires lengthy periods of landscape stability. As such, vast thicknesses of any fossil deposit may indicate relative tectonic, climatic and/or hydrological stability. However, it is essential that the morphological and geochemical characteristics of chemical sediments, as well as the environmental factors controlling their formation, are fully appreciated before they are used as evidence in palaeoenvironmental reconstruction. For example, when attempting to distinguish the significance of a calcrete within a sedimentary sequence, it is essential to determine whether it formed by pedogenic or non-pedogenic processes (see Wright, Chapter 2), since different processes of cementation may operate at different rates and represent different palaeohydrological conditions (e.g. Nash and Smith, 1998). This becomes even more critical when dealing with calcretes in the geological record (Pimentel et al., 1996) where fabrics may have been altered over time through processes of diagenesis and paragenesis.
Successful palaeoenvironmental interpretation is highly dependent upon the availability of representative and well-documented modern analogues. For many geochemical sediments, this is unproblematic as the processes involved in, and the controls upon, their formation are well understood. Studies of dripwater chemistry and environmental conditions within contemporary cave systems, for example, have greatly improved the hydrogeochemical interpretation of ancient speleothems (see Fairchild et al., 2006a,b; and Fairchild et al., Chapter 7). Similarly, Zhang et al. (2001) and Chen et al. (2004) have investigated the physico-chemical controls on contemporary carbonate precipitation at waterfalls, which has considerably enhanced our understanding of tufa and travertine formation (see Viles and Pentecost, Chapter 6). However, for materials such as silcrete (Nash and Ullyott, Chapter 4), there are virtually no representative modern equivalents, and debate continues over the precise environments under which they form (e.g. Summerfield, 1983b, 1986; Nash et al., 1994; Ullyott et al., 1998). Disagreements over the role of biological and physico-chemical mechanisms in the formation of rock varnish have also historically hindered their effective use as a palaeoenvironmental indicator, although recent developments will hopefully rectify this situation (see Liu, 2003; and Dorn, Chapter 8).
Geochemical sediments are increasingly being used as both relative and absolute age indicators. Duricrusts formed on palaeosurfaces as a result of pedogenic processes, for example, may represent important marker horizons and can, with considerable care, be used as a broad-scale correlative tool. However, it is the potential for absolute dating of geochemical sediments that is currently generating greatest interest. The dating of many CaCO3-cemented sediments has long been considered inappropriate due to concerns over whether the carbonate-cementing environment could be viewed as geochemically âclosedâ. Advances in the use of U-series dating mean that previously problematic materials such as calcrete can now be systematically dated (Kelly et al., 2000; Candy et al., 2004b, 2005). Similarly, the analysis and dating of microlaminations is permitting both palaeoenvironmental information and calibrated ages to be derived from rock varnish (see Liu et al., 2000; and Dorn, Chapter 8). These improvements may mean that such chemical sediments will, in the future, be used as routinely as speleothems (Fairchild et al., Chapter 7) and laminated lacustrine deposits (Verrecchia, Chapter 9) as chronometric and palaeoenvironmental tools.
Finally, many geochemical sediments are of major economic importance, both as sources of minerals and construction materials, and because of their potential impacts on human livelihoods through their influence upon soil properties and groundwater chemistry. In regions where alternative construction materials are scarce, geochemical sediments such as calcrete (Wright, Chapter 2), laterite (Widdowson, Chapter 3) and beachrock (Gischler, Chapter 11) may be used as building materials. For example, certain types of air-hardening laterite are widely employed as building bricks in Asia (Goudie, 1973), and calcrete was used as one of the main sources of road aggregate during the construction of the Trans-Kalahari Highway in Botswana in the late 1990s (Lawrance and Toole, 1984). In terms of mineral prospecting, evaporite sequences (Chivas, Chapter 10, and Goudie and Heslop, Chapter 12) provide economically significant sources of gypsum, nitrate, sulphate and borax, bauxite (Widdowson, Chapter 3) remains a key source of aluminium ore (e.g. Anand and Butt, 2003) and groundwater calcrete (Wright, Chapter 2) may contain significant concentrations of uranium (Carlisle et al., 1978; Carlisle, 1983).
Even where geochemical sediments are, in themselves, of little direct economic value, they may be of considerable utility in basin analysis, oil reservoir or aquifer characterisation and for locating economically important ore bodies (e.g. Smith et al., 1993; Abdel-Wahab et al., 1998; Butt et al., 2005). For example, chemical analyses of pedogenic calcretes are increasingly used as a gold-prospecting tool in southern Australia due to the preferential concentration of Au within profiles during bedrock weathering and cementation (Lintern et al., 1992); elevated levels of Au within the calcrete regolith may represent the near-surface expression of an area of concealed primary or secondary gold mineralisation (Lintern, 2002). Similarly, the upper ferruginous zone of lateritic profiles is frequently used as a sample medium for the detection of underlying Au ore bodies in southern and Western Australia (Butt et al., 2005). However, for these techniques to be successful, it is essential that sampling is undertaken with full regard to the local landform context (Craig, 2005), which requires that detailed regolithlandform mapping is carried out during the early phases of any mineral exploration programme (e.g. Hill et al., 2003). This ongoing work reinforces the premise behind this volume, namely that understanding the influence of landscape context upon the formation of any geochemical sediment is key to the successful exploitation of that precipitate or residual deposit. We are confident that as our understanding of the genesis of all geochemical sediments improves, then their economic value can only increase.
References
Abdel-Wahab, A., Salem, A.M.K. & McBride, E.F. (1998) Quartz cement of meteoric origin in silcrete and non-silcrete sandstones, Lower Carboniferous, western Sinai, Egypt. Journal of African Earth Sciences 27, 277â290.
Alley, N.F., Clarke, J.D.A., MacPhail, M. & Truswell, E.M. (1999) Sedimentary infillings and development of major Tertiary palaeodrainage systems of southcentral Australia. In: Thiry, M. & Simon-Coinçon, R. (Eds) Palaeoweathering, Palaeosurfaces and Related Continental Deposits. International Association of Sedimentologists Special Publication 27. Oxford: Blackwell Science, pp. 337â366.
Anand, R.R. & Butt, C.R.M. (2003) Distribution and evolution of âlateritesâ and lateritic weathering profiles, Darling Range, Western Australia. Australian Geomechanics 38, 41â58.
Barnes, L.C. & Pitt, G.M. (1976) The Mirackina Conglomerate. Quarterly Geological Notes of the Geological Survey of South Australia 59, 2â6.
Butt, C.R.M., Scott, K.M., Cornelius, M. & Robertson, I.D.M. (2005) Sample media. In: Butt, C.R.M., Robertson, I.D.M., Scott, K.M. & Cornelius, M. (Eds) Regolith Expression of Australian Ore Systems. Bentley, Western Australia: Cooperative Research Centre for Landscape Environments and Mineral Exploration, pp. 53â79.
Candy, I., Black, S. & Sellwood, B.W. (2004a) Complex response of a dryland river system to Late Quaternary climate change: implications for interpreting the climatic record of fluvial sequences. Quaternary Science Reviews 23, 2513â2523.
Candy, I., Black, S. & Sellwood, B.W. (2004b) Quantifying timescales of pedogenic calcrete formation using U-series disequilibria. Sedimentary Geology 170, 177â187.
Candy, I., Black, S. & Sellwood, B.W. (2005) U-series isochron dating of immature and mature calcretes as a basis for constructing Quaternary landform chronologies for the Sorbas Basin, southeast Spain. Quaternary Research 64, 100â111.
Carlisle, D. (1983) Concentration of uranium and vanadium in calcretes and gypcretes. In: Wilson, R.C.L. (Ed.) Residual Deposits: Surface Related Weathering Processes and Materials. Special Publication 11. London: Geological Society, pp. 185â195.
Carlisle, D., Merifield, P.M., Orme, A.R. & Kolker, O. (1978) The distribution of calcretes and gypcretes in southwestern United States and their uranium favorability. Based on a study of deposi...
Table of contents
- Cover
- Series
- Title
- Copyright
- List of Figures
- List of Tables
- List of Contributors
- Series Editorsâ Preface
- Acknowledgements
- Chapter One: Introduction: Geochemical Sediments in Landscapes
- Chapter Two: Calcrete
- Chapter Three: Laterite and Ferricrete
- Chapter Four: Silcrete
- Chapter Five: Aeolianite
- Chapter Six: Tufa and Travertine
- Chapter Seven: Speleothems
- Chapter Eight: Rock Varnish
- Chapter Nine: Lacustrine and Palustrine Geochemical Sediments
- Chapter Ten: Terrestrial Evaporites
- Chapter Eleven: Beachrock and Intertidal Precipitates
- Chapter Twelve: Sodium Nitrate Deposits and Efflorescences
- Chapter Thirteen: Analytical Techniques for Investigating Terrestrial Geochemical Sediments
- Chapter Fourteen: Geochemical Sediments and Landscapes: General Summary
- Index