
- 448 pages
- English
- ePUB (mobile friendly)
- Available on iOS & Android
Optical Processes in Semiconductors
About This Book
Based on a series of lectures at Berkeley, 1968–1969, this is the first book to deal comprehensively with all of the phenomena involving light in semiconductors. The author has combined, for the graduate student and researcher, a great variety of source material, journal research, and many years of experimental research, adding new insights published for the first time in this book.
Coverage includes energy states in semiconductors and their perturbation by external parameters, absorption, relationships between optical constants, spectroscopy, radiative transitions, nonradiative recombination, processes in pn junctions, semiconductor lasers, interactions involving coherent radiation, photoelectric emission, photovoltaic effects, polarization effects, photochemical effects, effect of traps on luminescence, and reflective modulation.
The author has presented the subject in a manner which couples readily to physical intuition. He introduces new techniques and concepts, including nonradiative recombination, effects of doping on optical properties, Franz-Keldysh effect in absorption and emission, reflectance modulation, and many others. Dr. Pankove emphasizes the underlying principle that can be applied to the analysis and design of a wide variety of functional devices and systems. Many valuable references, illustrative problems, and tables are also provided here.
Frequently asked questions
Information
ENERGY STATES IN SEMICONDUCTORS
1
1-A Band Structure
1-A-1 BANDING OF ATOMIC LEVELS
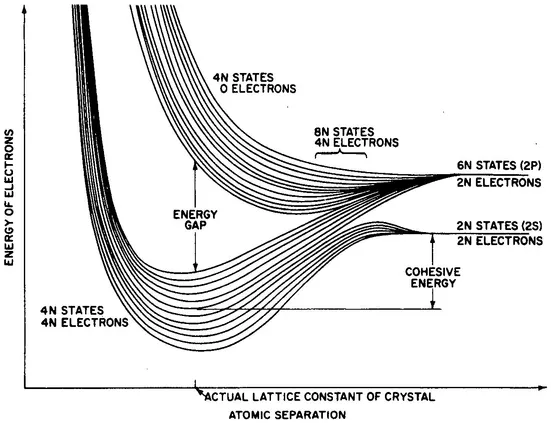
1-A-2 DISTRIBUTION IN MOMENTUM SPACE




Table of contents
- DOVER SCIENCE BOOKS
- Title Page
- Copyright Page
- Dedication
- PREFACE
- Table of Contents
- OPTICAL PROCESSES IN SEMICONDUCTORS
- ENERGY STATES IN SEMICONDUCTORS - 1
- PERTURBATION OF SEMICONDUCTORS BY EXTERNAL PARAMETERS - 2
- ABSORPTION - 3
- RELATIONSHIPS BETWEEN OPTICAL CONSTANTS - 4
- ABSORPTION SPECTROSCOPY - 5
- RADIATIVE TRANSITIONS - 6
- NONRADIATIVE RECOMBINATION - 7
- PROCESSES IN p-n JUNCTIONS - 8
- STIMULATED EMISSION - 9
- SEMICONDUCTOR LASERS - 10
- EXCITATION OF LUMINESCENCE AND LASING IN SEMICONDUCTORS - 11
- PROCESSES INVOLVING COHERENT RADIATION - 12
- PHOTOELECTRIC EMISSION - 13
- PHOTOVOLTAIC EFFECTS - 14
- POLARIZATION EFFECTS - 15
- PHOTOCHEMICAL EFFECTS - 16
- EFFECT OF TRAPS ON LUMINESCENCE - 17
- REFLECTANCE MODULATION - 18
- APPENDIX
- INDEX