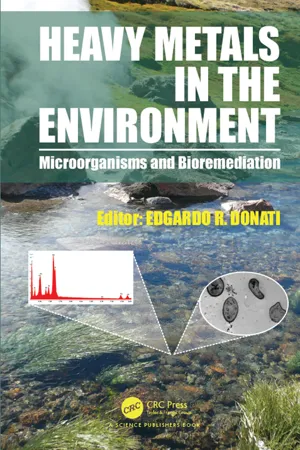
This is a test
- 324 pages
- English
- ePUB (mobile friendly)
- Available on iOS & Android
eBook - ePub
Book details
Book preview
Table of contents
Citations
About This Book
This book serves as a knowledge bank for researchers and graduate students in microbiology, chemistry, and environmental sciences, among others. It focuses on heavy metal in the environment and describes methodologies to immobilize and mobilize heavy metals. It also provides case studies which may be of particular interest to persons in industry.
Frequently asked questions
At the moment all of our mobile-responsive ePub books are available to download via the app. Most of our PDFs are also available to download and we're working on making the final remaining ones downloadable now. Learn more here.
Both plans give you full access to the library and all of Perlegoâs features. The only differences are the price and subscription period: With the annual plan youâll save around 30% compared to 12 months on the monthly plan.
We are an online textbook subscription service, where you can get access to an entire online library for less than the price of a single book per month. With over 1 million books across 1000+ topics, weâve got you covered! Learn more here.
Look out for the read-aloud symbol on your next book to see if you can listen to it. The read-aloud tool reads text aloud for you, highlighting the text as it is being read. You can pause it, speed it up and slow it down. Learn more here.
Yes, you can access Heavy Metals in the Environment by Edgardo R. Donati in PDF and/or ePUB format, as well as other popular books in Biological Sciences & Environmental Science. We have over one million books available in our catalogue for you to explore.
Information
PART I
INTERACTION METAL(LOID)S: MICROORGANISMS
CHAPTER 1
Microbial Communities and the Interaction with Heavy Metals and Metalloids
Impact and Adaptation
CINDEFI (CCT La Plata-CONICET, UNLP), Facultad de Ciencias Exactas, Universidad Nacional de La Plata, Calle 47 y 115, (1900) La Plata, Argentina.
* Corresponding author: [email protected]
1. Introduction
1.1 Heavy metals and their influence on microbial diversity
The millenary activity of extracting heavy metals (HM) is nowadays considered to be one of the main economic activities of the world with MĂŠxico, Chile, China and Australia being some of the countries with higher productions. Due to their particular properties, HM were and still are, used in many industries and production activities. Consequently, HM consumption has increased at a higher rate than population which has led to the generation and accumulation of tons of wastes. The inappropriate disposal of HM wastes has caused their mobility into the environment causing serious alterations in the ecosystems and triggering health problems in populations close to the polluted areas. The mobility of HM and metalloids into the environment also increases their distribution (especially in water systems) and bioavailability. The mobility and bioavailability of these pollutants are controlled by many chemical and biochemical processes such as precipitation/dissolution, sorption/desorption, complexation/dissociation and oxidation/reduction. The increase in HM and metalloids concentration in the environment directly affects microbial diversity, community structure and metabolic activity. It also causes the disappearance of susceptible species and the increase of tolerant species and/or the increase of the expression of resistance mechanisms (Zampieri et al., 2016).
1.2 Study of the impact of HM in microbial communities
The impact of heavy metals in the diversity of microbial communities has been studied for several years. The methods used have evolved with the passage of time. Culture dependent techniques are not quite suitable to study microbial diversity because only a small fraction of the microorganisms present in a sample can be successfully recovered in culture, even more if the sample contains any sort of contaminant such as heavy metals. Traditional molecular ecology techniques have been extensively used to characterize the microbial diversity in environments contaminated with heavy metals, either natural or from anthropogenic origin, such as mine tailings or industrial areas. Among the most commonly used techniques is DGGE (denaturating gradient gel electrophoresis) that involves the amplification of a short fragment of the 16S rRNA gene (aprox. 500 bp) of all the members of a microbial community that then are electrophoretically separated by sequence differences by the denaturizing effect of urea and formamide. In this way the band pattern of each sample reflexes the complexity of the microbial community. Moreover, the bands can be cut from the gel, the DNA eluded, re-amplified and sequenced to know the identity of the microorganisms present. An important advantage of DGGE is that it allows for a rapid comparison among different samples, for example the microbial community in a sample after and before being exposed to heavy metals. Another strategy that is used is the amplification of the whole 16S rRNA gene, cloning to construct a sequence library and sequencing the clones. The success of covering the entire microbial diversity of a sample depends on the number of clones sequenced, which is why it is recommended to do a DGGE run initially to assess the potential number of species present. Among the most modern approaches, the metaomics techniques allows for a much deeper study in several aspects of the community behavior.
Metagenomics, the massive sequencing of the microbial community DNA, can be applied to a specific gene, for example the 16S rRNA, or to the entire genetic material. 16S rRNA metagenomics studies produce millions of sequences, which are longer with the advances of sequencing technologies, that can be used in robust statistics analysis of microbial community structure and diversity and, by comparison of different samples, the changes suffered by any stress source.
Metatranscriptomics deals with the massive sequencing of the whole RNA of a microbial community and gives information on the genes that are expressed in certain conditions and time. In the same way metaproteomics reveals all the proteins produced by the community. Both, metatranscriptomics and metaproteomics, allow the comparison of RNA or proteins, respectively, in two different conditions, which is fundamental to understand the metabolic changes in a microbial community impacted by heavy metals.
A completely different approach was taken by Wang and co-worker (2010) who used microcalorimetry to assess and compare the toxic effect of heavy metals, such as As, Cu, Cd, Cr, Co, Pb, and Zn, on soil microbial activities and community. The samples of soil were supplemented with glucose, ammonium sulfate and each heavy metal. The metabolic activity was estimated by calculating certain thermodynamic parameters associated with growth rate, such as microbial growth rate constant, total heat evolution, metabolic enthalpy and mass specific heat rate. According to the authors these parameters can act as indicators of changes occurred in soil due to their exposure to heavy metals, and allow them to propose a ranking of toxicity (Cr > Pb > As > Co > Zn > Cd > Cu).
2. Heavy metalsâ resistance mechanisms
Metals and metalloids can be classified into three categories according to their biological roles and effects on microorganisms (Prabhakaran et al., 2016):
⢠Essential metals (Na, Ca, K, Mn, Mg, V, Fe, Cu, Co, Mo, Ni, Zn, and W): have recognized biological role, however, their ions become toxic if the concentration increases.
⢠Toxic metals (Ag, Sn, Cd, Au, Ti, Hg, Pb, Al and metalloids Ge, Sb, As, and Se): do not have a biological role and can interfere in cellular processes.
⢠Non-essential (Rb, Sr, Cs, and T): do not have a defined biological role and have no toxicity.
Essential metals function as co-factors for enzymes and stabilizers of protein structures and bacterial cell walls, and help maintaining osmotic balance. For instance, Fe, Cu, and Ni are involved in redox processes and Mg and Zn stabilize various enzymes and DNA through electrostatic forces. Also, Fe, Mg, Ni, and Co are a part of molecular complexes with a wide array of functions. Toxicity occurs through the displacement of essential metals from their native binding sites or through ligand interactions. Toxic metals bind with higher affinity to thiol groups and oxygen binding sites than essential metals do. Toxicity results from alterations in the conformational structure of nucleic acids and proteins and due to interference with oxidative phosphorylation and osmotic balance (Bruins et al., 2000).
Microorganisms are the oldest member of the living system and possess high adaptability strength to thrive in adverse conditions. They primarily respond to the changes in the environment by altering their genetic system or by transferring the genetic elements among other mechanisms for maintaining the structure and function of ecosystem (Das et al., 2016). Bacteria can use a set of direct or indirect mechanism in order to avoid the toxic effect of HM; for example:
Metal expulsion by a permeability barrier. Before entering the cell some HM can be retained by alterations in the cell wall or outer membrane to protect metal-sensitive internal components. Certain periplasmic proteins that are able to bind to specific metals have been described in different species. Giner-Lamia et al. (2015) studied a Cu-binding protein named CopM that is encoded in the CopMRS operon from the cyanobacterium Synechocystis sp. PCC 6803; the expression of the operon is activated by the product of CopMR when Cu ions are in the media, CopM bind Cu(I) in the periplasm and export it outside the cell avoiding the intracellular Cu accumulation (Fig. 1a). Similar proteins involved in Cu resistance were found in other microorganisms: CusF in E. coli (Franke et al., 2003), CueP in Salmonella typhimurium (Pontel and Soncini, 2009) and CopK in C. metallidurans (Mergeay et al., 2003). Also, the production of exopolysaccharides (EPS) by some microorganisms allows the retention of metal ions outside the cell. The presence of many functional anionic groups in EPS, such as carboxyl, phosphoryl, sulfhydryl, phenolic and hydroxyl groups, function as metal-binding sites with the possibility of cation exchange (Fig. 1b), physical sorption, complexation and/or precipitation of HM (More et al., 2014). Deschatre et al. (2015) studied an EPS produced by a marine bacteria which showed the maximum sorption capacities to be as high as 400 mg/g EPS and 256 mg/g EPS for Cu (II) and Ag (I), respectively, which are highly promising for bioremediation of these metals.
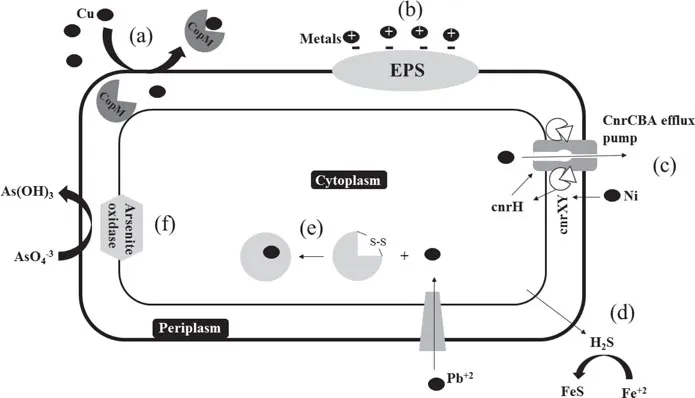
Figure 1. Overview of microbial HM resistance mechanisms. (a) Cu expulsion by the periplasmic Cu-binding protein CopM. (b) HM cations retention by anionic groups of EPS. (c) Ni expulsion from the cell by a specific efflux pump. (d) Extracellular Fe precipitation as FeS by SRB. (e) Intracellular Pb sequestration by the Pb-binding protein pbrD. (f) Oxidation of arsenite to a less toxic form by the arsenite oxidase.
Active transport for expulsion of the metal from the cell. Microorganisms use active transport mechanisms to export toxic metals from their cytoplasm. These mechanisms can be encoded in the chromosome or on plasmids. Non-essential metals normally enter the cell through normal nutrient transport systems but are rapidly exported. These efflux systems, that can be ATP dependent or not, are highly specific for the cation or anion they export (Bruins et al., 2000). Ni resistance is generally mediated by active transport; Grass et al. (2000) reported the mechanisms of the operon cnrYHXCBAT founded in Cupriavidus metallidurans which encodes the highly efficient CnrCBA efflux pump that is activated by the sigma factor cnrH and the membrane-bound proteins cnrY and cnrX at ÎźM concentrations of Ni. The mechanism is illustrated in Fig. 1c, when periplasmic Ni binds to cnrX, the anti-sigma factor cnrXY release cnrH which indirectly initiates the transcription of the CnrCBA efflux pump, necessary to expel Ni from the cell. In the case of Cu, one of the main systems of expulsion mediated by transporters, is that codified by the operon copYABZ, where copA and copB are P-type ATPases, also able to transport other metals such as Ag, Zn, and Cd. Besaury et al. (2013) reported high abundances of the ATPase CopA were found in Cu-contaminated Chilean marine sediments.
Extracellular metal sequestration. Microorganisms produce and expel metabolites such as phosphate, glutathione, oxalate, sulfur, among others that can bind metal and the complex metal-metabolite cannot pass through the cell membrane, thus decreasing or avoiding the absorption and intracellular harm. Sulfate reducing bacteria (SRB) that generate hydrogen sulfide through dissimilatory sulfate reduction are a great example of this mechanism; sulfide reacts with certain metals such as Cu, Zn, or Fe (Fig. 1d) forming insoluble precipitates thus decreasing the concentration of bioavailable metal. This mechanism could be critical for certain species. For instance when an acidophilic metal tolerant Desulfosporosinus was isolated from an abandoned gold mine highly contaminated with arsenic, the strain could not grow at very low concentrations of As even though the major tolerance mechanisms were present in its genome. The authors propose that As, could be precipitated as sulfides by SRB; in fact two different arsenic sulfides were found in the sediments samples of the tailing, thus, lowering the bioavailable concentration of As in the environment (Mardanov et al., 2016).
Intracellular metal sequestration. Bacteria are capable to complex metals and accumulate them in the cytoplasm. Complexants include glutathione and bacterial metallothioneins, also, Pb and Hg may accumulate in polyphosphate inclusions (Guillan, 2016). JarosĹawiecka and Piotrowska-Seget (2014) described the mechanisms of Pb resistance in C. metallidurans CH34; this species has the pbrABCD operon that encoded a group of proteins involved in Pb resistance. In the presence of Pb+2pbrR, a regulatory protein, induced the expression of pbr, one of the product of this transcription is pbrD, an intracellular Pb binding protein in which the binding-site is rich in cysteine residues and also bears a large number of proline and serine residues that sequester Pb reducing its toxic effects (Fig. 1e). Similarly the mer operon is induced by the presence of Hg+2; merR is the activator of the expression and merC is the protein involved in the transport and accumulation of Hg. Some bacteria have the ppk gene that encodes polyphosphate kinase involved in polyphosphate biosynthesis. These negatively charged orthophosphate polymers are capable of binding Hg ions among others (Das et al., 2016).
Detoxification/Transformation to a less toxic form. It includes processes such as oxidation or reduction, methylation or volatilization. Detoxification of As is one of the most clear examples; As(V) is less toxic and less mobile than As(III) and thus some microorganisms use oxidation as a resistance strategy. The ArsC protein is involved in the oxidation of As(III) to As(V) for the purpose of detoxification only. But some chemolitho...
Table of contents
- Cover
- Half Title
- Title Page
- Copyright Page
- Table of Contents
- Preface
- PART I INTERACTION METAL(LOID)S: MICROORGANISMS
- PART II STRATEGIES OF BIOREMEDIATION
- PART III BIOREMEDIATION OF RELEVANT METAL(LOID)S
- PART IV FIELD APPLICATIONS
- Index