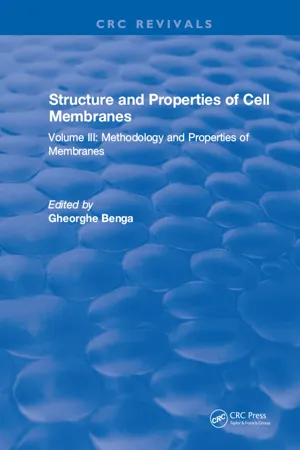
eBook - ePub
Structure and Properties of Cell Membrane Structure and Properties of Cell Membranes
Volume III
This is a test
- 298 pages
- English
- ePUB (mobile friendly)
- Available on iOS & Android
eBook - ePub
Structure and Properties of Cell Membrane Structure and Properties of Cell Membranes
Volume III
Book details
Book preview
Table of contents
Citations
About This Book
This book provides in-depth presentations in membrane biology by specialists of international repute. The volumes examine world literature on recent advances in understanding the molecular struc-ture and properties of membranes, the role they play in cellular physiology and cell-cell interactions, and the alterations leading to abnormal cells. Illustrations, tables, and useful appendices com-plement the text. Those professionals actively working in the field of cell membrane investigations as well as biologists, biochemists, biophysicists, physicians, and academicians, will find this work beneficial.
Frequently asked questions
At the moment all of our mobile-responsive ePub books are available to download via the app. Most of our PDFs are also available to download and we're working on making the final remaining ones downloadable now. Learn more here.
Both plans give you full access to the library and all of Perlegoâs features. The only differences are the price and subscription period: With the annual plan youâll save around 30% compared to 12 months on the monthly plan.
We are an online textbook subscription service, where you can get access to an entire online library for less than the price of a single book per month. With over 1 million books across 1000+ topics, weâve got you covered! Learn more here.
Look out for the read-aloud symbol on your next book to see if you can listen to it. The read-aloud tool reads text aloud for you, highlighting the text as it is being read. You can pause it, speed it up and slow it down. Learn more here.
Yes, you can access Structure and Properties of Cell Membrane Structure and Properties of Cell Membranes by Gheorghe Benga in PDF and/or ePUB format, as well as other popular books in Biological Sciences & Cell Biology. We have over one million books available in our catalogue for you to explore.
Information
Chapter 1
QUANTUM CHEMICAL APPROACH TO STUDY THE MECHANISMS OF PROTON TRANSLOCATION ACROSS MEMBRANES THROUGH PROTEIN MOLECULES
TABLE OF CONTENTS
I. | Introduction | ||
II. | Methods | ||
III. | Applications | ||
A. | Accuracy of the Calculations | ||
B. | Angular Distortions | ||
C. | External Influences | ||
1. | pH | ||
2. | External Charges | ||
D. | Coupling of Multiple Transfers | ||
IV. | Conclusions | ||
Acknowledgments | |||
List of Abbreviations | |||
References |
I. INTRODUCTION
The last several decades have seen an evolution of the concept of cell membranes from simple physical separations between âinsideâ and âoutsideâ to very active regions where many diverse biochemical reactions are taking place.1 In fact, biomembranes have now been identified as the location where the cell generates and utilizes a great deal of its energy. For example, the generation of ATP via both photosynthetic and oxidative phosphorylation takes place at the membrane of the thylakoid or mitochondria, respectively.2, 3, 4, 5, 6, 7, 8, 9, 10, 11, 12, 13, 14 Research has further provided evidence that a common feature in biological energy transduction processes is the transport of protons across the biomembrane. Mitchellâ15, 16, 17 chemiosmotic hypothesis describes the coupling of energy transduction to a âprotonmotive forceâ across the membrane. This force is composed of two parts: an electric field generated by an excess of charge on one side of the membrane, and a pH gradient resulting from a difference in proton concentrations. The transport of protons across the biomembrane and âdownâ the pH gradient supplies the driving force for ATP synthesis in much the same way that passage of electrons through a filament provides the energy necessary to cause a lightbulb to glow. Indeed, the storage of electric energy in a charged capacitor provides a convenient analogy to the utilization by the cell of a pH gradient in a membrane as a storehouse of chemical energy.
Since the proton translocation provides the energy necessary for phosphorylation, it is important to determine the molecular mechanism of the former process including the pathway that the protons take through the membrane. The highly hydrophobic nature of the lipid bilayer1 provides a very hostile environment for the electrically charged protons. On the other hand, the hydrophilic domains found in proteins suggest that an alternate and more favorable route for the protons might lie directly through transmembrane proteins which are imbedded in and completely traverse the lipid bilayer. This pathway is indicated schematically by the dashed line in Figure 1. An example is provided by mitochondrial oxidative phosphorylation where the F0 segment of H+-ATPase appears to function as a proton channel.7, 8, 9, 10, 11, 12, 13, 14,18 Studies have shown also that bacteriorhodopsin, the major protein contained in the purple membrane of Halobacterium acts as a light-driven proton pump in which the protons being translocated pass directly through the protein.19, 20, 21, 22, 23, 24
Three different mechanisms have been proposed by which the protons might be translocated across the thickness of the membrane. The first involves the motion of a proton-binding group of the protein back and forth between the two membrane surfaces. This is extremely unlikely, however, because large-scale conformational changes are not expected in the well-ordered protein crystal lattice of bacteriorhodopsin.22,23 Temperature dependence studies suggest that mobile proton carriers are not present in ATPase either.25
A second and more attractive mechanism for proton migration takes advantage of the large numbers of hydrogen bonds normally found within protein molecules. This mechanism, originally suggested by Eigen26,27 and Onsager28,29 and further elaborated by Nagle and Morowitz,30 presupposes the existence within the protein of a continuous hydrogen-bond chain of protein residues, including perhaps several water molecules as well. This chain, indicated by the dashed line in Figure 1, is illustrated in greater detail in Figure 2. Although the H-bonding groups are represented here by hydroxyls which occur in the side chains of serine, threonine, and tyrosine, it is emphasized that this is by no means a requirement. Other H-bonding groups which might participate in the chain include the amino group of lysine, carboxyl of aspartic acid, and the amide groups which occur in the polypeptide backbone. To operate as a proton conduit, the chain would necessarily be oriented such that within the confines of the protein framework, it extends from the interior surface of the membrane to the exterior. In general, at least 20 groups would be needed for a chain of the proper length;30 however, for purposes of clarity, only five residues are included in Figure 2. Initially, the chain is in the OHâOHâOH configuration wherein all hydroxyl groups âpoint to the rightâ, as in Figure 2a. A proton from the internal medium approaches and binds to the leftmost oxygen, leaving this atom with an excess proton and formal positive charge. As shown by the curved arrow in Figure 2a, the proton initially bound covalently to this oxygen and H-bonded to the second oxygen of the chain, then transfers across to the latter atom. This hop is followed by similar transfers of each hydrogen atom from one oxygen to the next along the chain. As indicated on the right of Figure 2a, the hopping terminates with the expulsion of the last hydrogen into the medium external to the membrane. The entire process to this point has resulted in two net effects. A single proton has been transported from one side of the membrane to the other and the chain has been changed from its original OHâOHâOH configuration to HOâHOâHO, as illustrated in Figure 2b. Transformation of the chain to its original configuration and ready to transport a second proton is accomplished by rotation of each hydroxyl group about its respective RâO bond.
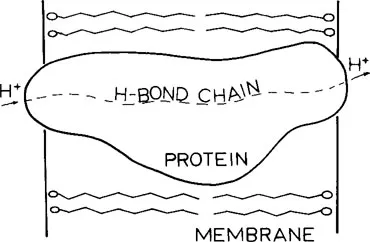
FIGURE 1. Schematic diagram of a protein embedded in the lipid bilayer of a membrane. The broken line represents a path of protons through the protein via a H-bond chain of protein residues.
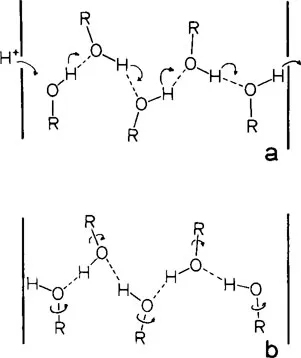
FIGURE 2. Proton conduction mechanism. The interfaces of the membrane with aqueous regions are indicated by the thick vertical lines. The curved arrows in a represent hops of protons between O atoms. Rotations of the hydroxyl groups about the RâO bonds are indicated by the arrows in b.
The above mechanism for proton transport has been postulated for membrane proteins such as bacteriorhodopsin23,31, 32, 33, 34, 35, 36 and ATPase.25,37 Similar processes have been shown to operate as a proton conduction device in ice28,29 where the hydrogen-bonded chains are formed exclusively from water molecules. In liposomes which contain no proteins, water molecules in the bilayer appear to organize into hydrogen-bonding strands which bridge the lipophilic region of the bilayer and transport protons as described above.38 Similar processes function also in imidazole,39 a model of the hydrogen bonding amino acid histidine, as well as in inorganic substances.40,41 The existence of the required continuous chains of hydrogen bonds in proteins is becoming increasingly well documented. The recently obtained amino acid sequence and diffraction data of bacteriorhodopsin34,36 indicate a chain of hydrogen bonds in the protein which completely spans the membrane. The importance of this chain to the functioning of bacteriorhodopsin is emphasized by recent evidence34 that a number of charged residues form hydrogen-bonded pairs within the bilayer or âburiedâ segment of the protein and not in the aqueous portion as would ordinarily be more favorable energetically. In murein-lipoprotein of Escherichia coli42 an extended set of interconnecting hydrogen bonds of total approximate length 55 A has been suggested. Us...
Table of contents
- Cover
- Title Page
- Copyright Page
- Table of Contents
- Chapter 1 Quantum Chemical Approach to Study the Mechanisms of Proton Translocation Across Membranes Through Protein Molecules
- Chapter 2 Monomolecular Films as Biomembrane Models
- Chapter 3 Planar Lipid Bilayers in Relation to Biomembranes
- Chapter 4 Relation of Liposomes to Cell Membranes
- Chapter 5 Reconstitution of Membrane Transport Systems
- Chapter 6 Structure-Function Relationships in Cell Membranes as Revealed by X-Ray Techniques
- Chapter 7 Structure-Function Relationships in Cell Membranes as Revealed by Spin Labeling EPR
- Chapter 8 Structure and Dynamics of Cell Membranes as Revealed by NMR Techniques
- Chapter 9 The Effect of Dietary Lipids on the Composition and Properties of Biological Membranes
- Index