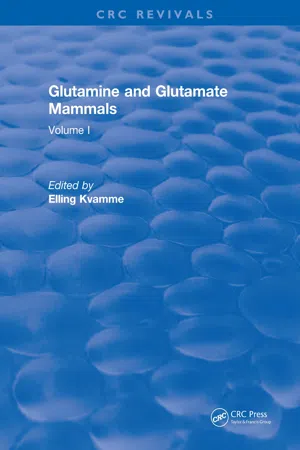
This is a test
- 280 pages
- English
- ePUB (mobile friendly)
- Available on iOS & Android
eBook - ePub
Book details
Book preview
Table of contents
Citations
About This Book
Based on the joint effort of a great many top scientists, this book covers most aspects of the metabolism and function of glutamine and glutamate in mammals.
Frequently asked questions
At the moment all of our mobile-responsive ePub books are available to download via the app. Most of our PDFs are also available to download and we're working on making the final remaining ones downloadable now. Learn more here.
Both plans give you full access to the library and all of Perlego’s features. The only differences are the price and subscription period: With the annual plan you’ll save around 30% compared to 12 months on the monthly plan.
We are an online textbook subscription service, where you can get access to an entire online library for less than the price of a single book per month. With over 1 million books across 1000+ topics, we’ve got you covered! Learn more here.
Look out for the read-aloud symbol on your next book to see if you can listen to it. The read-aloud tool reads text aloud for you, highlighting the text as it is being read. You can pause it, speed it up and slow it down. Learn more here.
Yes, you can access Glutamine and Glutamate Mammals by Elling Kvamme in PDF and/or ePUB format, as well as other popular books in Medicine & Alternative & Complementary Medicine. We have over one million books available in our catalogue for you to explore.
Information
Chapter 1
GLUTAMATE AND GLUTAMINE IN MAMMALS: AN OVERVIEW
William J. Nicklas
Glutamate and glutamine are key amino acids in mammalian intermediary metabolism, intimately associated with aerobic metabolism via the tricarboxylic acid cycle and with ammonia metabolism. In addition they are involved in the synthetic pathways of numerous biologically important compounds.1 Research in various aspects of the metabolism and function of these amino acids has been continuous since the earliest days of modern biochemical studies.2 The various contributions to this monograph attest to the vitality and conceptual depth of current research efforts. The direction of these studies has traced a path through the traditional biochemical methodologies and now encompasses other powerful techniques such as immunohistochemistry and molecular biology.
What is emerging from these multidisciplinary studies on different organ systems is a pattern of greater complexity and heterogeneity than previous work might have suggested. Not only does one find the expected intracellular and interorgan diversity, but intercellular heterogeneity is becoming a more commonly accepted phenomenon. Nowhere is this complexity and heterogeneity more apparent, nor has it been more intensely studied, than in the nervous system.3,5 In the central nervous system (CNS), glutamate and its decarboxylation product, 4-aminobutyrate (GABA), may well function as important neurotransmitter substances.6 Thus superimposed on the “normal” role of glutamate and glutamine in cellular metabolism with its accompanying transport systems and enzymes is the further complication of specific membrane-associated receptor proteins and the associated ion-channel systems. Furthermore, glutamate and related neuroexcitatory substances are also potentially neurotoxic. The pharmacological and toxicological consequences of multiple receptor types for excitatory dicarboxylic amino acids are only now being understood and exploited.7,8 It is clear from this that exquisite regulatory mechanisms must be present to separate these various functions of ubiquitously distributed amino acids such as glutamate and glutamine. The homeostatic regulation of the neuroactive amino acids is associated with a heterogeneity of enzyme distribution among the neurons and glial cells that make up the nervous system.9 Most current evidence would suggest that transmitter pools of glutamate are formed in glutamatergic synaptic endings from glutamine via the activity of phosphate-activated glu-taminase [EC 3.5.1.2], which catalyzes the hydrolytic cleavage of glutamine to glutamate and ammonia. It is doubtful that this enzyme is localized principally in such neurons but there may be some relative enrichment. Regulation probably occurs via product inhibition and presence of other effectors, such as Ca2+.10,11 Glutamine synthesis in the brain appears to be principally associated with the activity of glutamine synthetase [EC 6.3.1.2] which catalyzes the ATP-dependent amidation of glutamate.12 This also serves to remove ammonia from the circulation since the urea cycle is inoperative in brain. Early experiments in which animals were injected with radioactive precursors suggested that glutamine synthesis in the brain was compartmented. For example, when rats were injected intracisternally (thus avoiding problems with the blood-brain barrier) with 14C-glutamate and the total pools of brain glutamate and glutamine acid-extracted, it was found that within minutes the radiospecific activity of the total glutamine was severalfold higher than that of the total brain glutamate.13 This indicated that the radioactive extracellular glutamate was metabolized in a biochemical compartment in which glutamine was synthesized before the radioactive glutamate could mix with the bulk of tissue glutamate. Based on a great deal of similar in vivo and in vitro data, Balazs et al.14 suggested the “compartment” in which glutamine was actively synthesized was the astroglial cells of the brain. The subsequent immunohistochemical localization of glutamine synthetase in astrocytes in the brain15 has definitively shown this interpretation based on biochemical studies to be correct. These findings immediately suggest problems of regulation of transport and metabolism which would not be so obvious in a less complex model. However, this complexity also allows for the separate regulation of the role of these amino acids in “normal” metabolism and their possible role in neurotransmission.
From these studies emerged a concept of a “glutamine cycle” between glia and nerve endings.9,16 In this hypothesis, glutamate and GABA levels within their respective nerve terminals are maintained by glutamine derived from the extracellular space which in turn can be replenished by synthesis in the glia via glutamine synthetase. In a series of studies we attempted to answer the question as to whether this really occurred.9 One consistent finding that emerged from the many earlier studies done with radioactive precursors of glutamate/glutamine was that radioactive acetate (either 14C- or 3H-labeled) is preferentially metabolized in the glutamine-synthesizing compartment of the brain, i.e., the astrocyte, whereas radioactively labeled glucose is metabolized in all compartments.16 Numerous studies have shown that when various CNS preparations are allowed to metabolize labeled glucose and/or acetate and then efflux of synaptic contents initiated by depolarization stimuli, there is a preferential efflux of glucose-labeled glutamate.9,17,18 In a series of experiments,9 tissue slice preparations from rat brains were incubated with a mixture of 14C-glucose and 3H-acetate to label endogenous pools of amino acids. The alkaloid veratridine was used to cause the efflux of synaptic glutamate and GABA. The proposed flow pathway from glutamine synthesis in glia to glutamine utilization in the nerve endings was blocked by using 6-diazo-5-oxo-L-norleucine (DON), an inhibitor of glutaminase, or L-methionine sulfoximine, an inhibitor of glutamine synthetase. These treatments decreased the veratridine-induced release of acetate-labeled glutamate and GABA preferentially. Therefore, glutamate and GABA labeled from radioactive acetate metabolism (i.e., from glial metabolism) did indeed enter transmitter pools of these amino acids via glutamine.9 Moreover, the contribution of glutamine metabolism to the maintenance of transmitter amino acid levels seemed to be quantitatively important. The above studies were performed with in vitro preparations. Rothstein and Tabakoff19 have come to a similar conclusion after in vivo inhibition of glutamine synthetase. Methionine sulfoximine was administered to rats intraventricularly and the glu-tamergic system of the striatum was studied. Striatal glutamine synthetase activity and glutamine levels were decreased substantially for days with no change in total glutamate levels. In addition, the Ca2+-dependent, K+-stimulated release of glutamate from striatal slices of treated animals was diminished by 50%. When glutamine was present in the perfusion medium in which release was measured, there was no difference in glutamate efflux between slices from control or treated animals. The same authors had previously reported that treatment of rats with intraventricular methionine sulfoximine decreased the activity of dopaminergic terminals in the striatum.20 This is of physiological interest because it has been suggested that corticostriatal glutamergic inputs presynaptically facilitate the release of dopamine from nigrostriatal nerve endings. At the present time, there is every reason to conclude that the “glutamine cycle” is operative in the CNS and is of quantitative and qualitative importance in the regulation of glutamate homeostasis.
When the first studies on amino acid metabolism in the brain were reported 2 decades ago, there was a good deal of skepticism in the traditional biochemical community. Some of this attitude was probably due to the perception of a relative homogeneity of amino acid metabolism in other organs, for example, in the liver which was the benchmark for comparison. Another important reason, perhaps, was a lack of awareness of how to properly interpret studies with tracer-radioactive precursors and the role played by high affinity active transport systems for substances such as glutamate when tracer amounts are utilized in such studies. In any case, doubts were slowly buried under an ever-increasing amount of data culminating in the immunohistochemical localization studies. In the meantime, the perception of the homogeneity of glutamate metabolism in organs such as the liver has been replaced by more recent, broadening concepts of its heterogeneity.
The early studies with isotopically labeled precursors administered intravenously to animals also suggested a compartmentation of glutamate/glutamine/ammonia metabolism in other organs such as the liver.13 This was not pursued at the time. It is now clear that ammonia metabolism in the liver is heterogeneous. Glutamine synthetase appears to be localized in a small layer of perivenous cells21 which results in a heterogeneity in glutamine and ammonia metabolism as studied by steady-state anterograde and retrograde perfusion experiments.22 Thus, there is a periportal location of urea synthesis and a perivenous location for glutamine synthesis in the liver. Each of these processes is subject to regulation and the resulting studies have been most fruitful in better understanding ammonia metabolism in the liver and its response to various physiological effectors.23 Hãussinger22 has proposed an intercellular “glutamine cycle” operating in the liver during ureogenesis which is to some extent reminiscent of the “glutamine cycle” of the brain. The disparate distribution of glutamine synthetase and the resulting heterogeneity of ammonia metabolism also impacts on the in situ activity of other enzymes which may be more homogeneously distributed, such as glutamate dehydrogenase [EC 1.4.1.3], an oxidoreductase which catalyses the NAD(P)-dependent deamination of glutamate to 2-oxoglutarate as well as the reverse reaction, the amination of 2-oxoglutarate to glutamate. The direction of the reaction can be different in different organs and under different conditions within the same organ. In the brain, the contribution of this enzyme to amino acid metabolism is not well understood but is under active investigation.24 Studies in the brain might well profit from what has been found in experiments with other organs such as the liver. As pointed out by Sies and Hãussinger,23 ammonia production from catabolism of endogenous sources implies an increased oxidative deamination of glutamate by glutamate dehydrogenase in the periportal lobule of the liver. Conversely, perivenous glutamine synthesis from ammonia released from periportal hepa-tocytes requires increased glutamate formation by glutamate dehydrogenase to maintain glutamate levels. Here again there is an interesting analogy to studies in the nervous system. Berl et al.13,25 in their pioneering studies on 15N-NH3 metabolism in the brain found an increased synthesis of glutamate which occurred via glutamate dehydrogenase when glutamine synthesis was stimulated by increased ammonia levels. Concomitantly, to maintain 2-oxoglutarate levels, fixation of carbon dioxide via pyruvate carboxylase was also stimulated. The astrocyte is fixed as the site where these effects occur by the fact that 14C-HC03 behaves like acetate as a glutamine precursor in vivo25 and in vitro,26 and pyruvate carboxylase appears to ...
Table of contents
- Cover
- Half title
- Title Page
- Copyright Page
- Preface
- THe Editor
- Contributors
- Table of Contents
- Chapter 1 Glutamate and Glutamine in Mammals
- ENZYMES IN GLUTAMINE AND GLUTAMATE METABOLISM
- EXTRANERVOUS METABOLISM OF GLUTAMINE AND GLUTAMATE
- INDEX