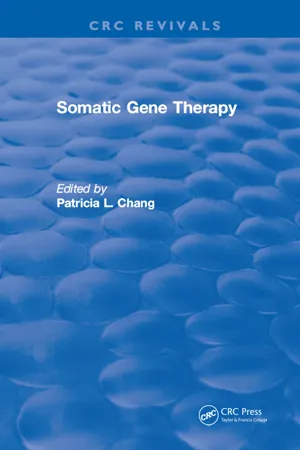
This is a test
- 313 pages
- English
- ePUB (mobile friendly)
- Available on iOS & Android
eBook - ePub
Somatic Gene Therapy
Book details
Book preview
Table of contents
Citations
About This Book
As human gene therapy becomes a clinical reality, a new era in medicine dawns. Novel and innovative developments in molecular genetics now provide opportunities to treat the genetic bases of diseases often untreatable before. Somatic Gene Therapy documents these historical clinical trials, reviews current advances in the field, evaluates the use of the many different cell types and organs amenable to gene transfer, and examines the prospects of various exciting strategies for gene therapy.
Frequently asked questions
At the moment all of our mobile-responsive ePub books are available to download via the app. Most of our PDFs are also available to download and we're working on making the final remaining ones downloadable now. Learn more here.
Both plans give you full access to the library and all of Perlegoâs features. The only differences are the price and subscription period: With the annual plan youâll save around 30% compared to 12 months on the monthly plan.
We are an online textbook subscription service, where you can get access to an entire online library for less than the price of a single book per month. With over 1 million books across 1000+ topics, weâve got you covered! Learn more here.
Look out for the read-aloud symbol on your next book to see if you can listen to it. The read-aloud tool reads text aloud for you, highlighting the text as it is being read. You can pause it, speed it up and slow it down. Learn more here.
Yes, you can access Somatic Gene Therapy by P.L. Chang in PDF and/or ePUB format, as well as other popular books in Biological Sciences & Genetics & Genomics. We have over one million books available in our catalogue for you to explore.
Information
1 | Overview |
Gene therapy is a novel form of molecular medicine that will have a major impact on human health in the next century. Although the advent of recombinant DNA technology in modern medicine will allow fetal genetic screening and genetic counseling, the vast majority born with disease are likely to be helped by gene therapy approaches. The scope and definition of gene therapy has expanded in the past few years. In addition to the possibility of correcting inherited genetic disorders like cystic fibrosis, hemophilia, and familial hypercholesterolemia, gene therapy approaches are being used to combat acquired diseases like cancer, AIDS, Parkinsonâs disease, Alzheimerâs disease, and infectious diseases. At present, germ line gene therapy is not being contemplated due to the complex technical and ethical issues. The scientific community is interested in pursuing somatic cell gene therapy, which is exclusively for the benefit of the individual and cannot be passed on to the succeeding generation. The minimum requirement for gene therapy is sustained production of the therapeutic gene product without any harmful side effects.1, 2, 3, 4, 5
I. THE SOMATIC TISSUE
There are a number of somatic tissues that have been used to transfer genes, but the precise choice of the tissue will be dictated by the nature of the disease. Bone marrow (see Chapters 2, 3, and 13) has been a favorite target because of the possibility of introducing the foreign gene in the pluripotent stem cell, but it has not been easy to obtain either therapeutic levels or sustained amounts of the foreign protein.6 Several innovations using specific growth factors and lymphokines will improve the availability of stem cells, and therefore allow sustained production of the foreign protein. Many researchers have also taken advantage of differentiated hematopoietic cells like circulating T cells, lymphocytes, etc., for gene expression. Due to extensive knowledge in handling primary fibroblast cells, many investigators have used fibroblasts (Chapter 4) as a choice tissue for introducing foreign genes where the therapeutic product can be secreted in the plasma, e.g., hemophilia proteins.7 The big drawback to date has been the lack of sustained production of the foreign protein, primarily due to âshut offâ of the transgene.8,9 Lack of appropriate promoters-enhancers specific for fibroblasts is partly responsible for the lack of progress in successfully using fibroblasts. Due to extensive advances in engineering of artificial skin in vitro for grafting at burn sites, keratinocytes (Chapter 6) offer a very viable target for introducing genes where the therapeutic product is secreted, but unfortunately, poor vascularization of keratinocytes has hampered their use. Nearly 40% of body weight is made up of muscle tissue (Chapter 8) and, therefore, it is an attractive target. Furthermore, myoblasts can be cultured in vitro and transplanted in the muscle to fuse with the existing myofibers. Muscle cells can also be directly infected and are very well vascularized. Not surprisingly, many investigators have relied on the use of muscle cells for producing therapeutic proteins.10 A very large number of metabolic diseases involve proteins synthesized in the liver (Chapter 5). Because liver is not an actively dividing tissue, usually ex vivo approaches have been employed. Hepatocytes obtained by partial hepatectomy have been transduced with foreign genes and then perfused into the liver. Recently, direct in vivo gene delivery approaches have also been used.11,12 Because of their close proximity to vasculature, many investigators have used endothelial cells (Chapter 7) to introduce genes either ex vivo or through a catheter in vivo. However, a major limitation of endothelial cells is their relatively small number, which renders them unlikely to produce therapeutic levels of the foreign gene products. The lack of postmitotic activity of neuronal cells has hindered the use of neuron/glia (Chapters 9, 10, and 14) for introducing foreign gene, but the advent of new vectors that may not require dividing cells should allow this problem to be overcome.
There are many other cell types, e.g., cardiac myocytes, lung air valves, macrophages, etc. but they have not been explored as extensively and, therefore, the discussion in this book has been confined to the commonly used cell types.
II. THE DELIVERY SYSTEM
Although a wide variety of somatic tissues has been identified for delivering genes, the major hurdle is still the mode of delivery of the therapeutic genes. Briefly, the currently available gene delivery approaches can be divided into two main types.
A. PHYSICO-CHEMICAL METHODS
This approach involves DNA transfection with calcium phosphate, DEAE dextran, cationic lipids, etc., direct DNA injection with or without the aid of cationic lipids, electroporation, ballistic guns delivering gold-coated DNA particles, microinjection, liposomes, and receptor-mediated gene transfer (see Chapter 11). All these approaches have benefits, but it is fair to say they are not very successful by and large in delivering large and sustained amounts of therapeutic gene product. For instance, an average individual secretes 5 Îźg/ml of factor IX protein in the plasma, and the present methodology is just not quite up to par.
B. BIOLOGICAL VECTORS
For any large-scale introduction of a biological entity to cells, the most likely approach will employ the use of viral vectors. Since high-titer viruses (up to 106 to 1013 virus particles/ml) can be obtained (depending on the type of virus), it is easy to infect large numbers of cells. Additionally, pluripotential stem cells, which are rare (1 per 103 to 104 cells), can also be infected with high-titer viruses. Although there are many viral vectors, attention has been focused mainly on three types of viral vectors.
1. Retroviral Vectors
They have been used most extensively in gene therapy approaches1, 2, 3, 4, 5, 6,14 because of ease of manipulation, integration in the host chromosome and thus sustained expression, ability to infect a wide variety of cell types from a variety of species, ability to accommodate foreign genes ranging from 1 to 7 kb, and ability to generate replication deficient viruses because the proteins necessary for viral replication can be provided in trans. Limitations: (1) The major limitation of retroviruses is their inability to infect postmitotic cells, which limits their use for most in vivo gene delivery approaches. Usually the somatic tissue needs to be manipulated in vitro. The ability of some lentiviruses to infect nondividing cells offers some hope of overcoming this limitation. (2) Although titers of 105 to 106 can be generally achieved, higher titers will be required for attaining therapeutic levels. In this regard the recent availability of recombinant retroviruses containing vesicular stomatitis virus (VSV) glycoprotein allows concentration of the recombinant retrovirus by centrifugation to obtain titers of 108â9 virus particles/ml. (3) There is some risk of integration of retroviruses in the vicinity of a proto-oncogene and may cause neoplasia.
2. Adenoviral Vectors
Adenovirus types 2 and 5 (Ad2 and Ad5) cause respiratory disease in humans but belong to a subclass of adenovirus that is not associated with human malignancies.15 The safety of recombinant adenovirus is supported by the extensive and effective use of live adenovirus vaccines in human population, mostly military recruits in the U.S. The genome of adenovirus is composed of linear, double-stranded DNA of approximately 36 kb in length with short inverted terminal repeats (ITR) at each end of the genome required for viral DNA replication. Adenoviruses have a lytic cycle, and infection is carried out by entry into the cell by as yet unidentified receptors, followed by entry into endosome, release in the cytoplasm with loss of accompanying structural proteins, and migration of the viral DNA to the nucleus, where it is retained as a linear structure. The initial hesitation of the relatively large size of adenoviruses (36 kb) has largely been overcome by very successful use of adenoviral vectors for both ex vivo and in vivo gene delivery (for a review see Reference 16). The major advantage of adenoviral vectors are (1) high titer (1011 to 1012 virus particle/ml) or recombinant viruses, (2) ability to infect postmitotic cells, (3) ability to infect a wide variety of cell types, (4) ability to accommodate up to 10 kb of foreign DNA, and (5) they are known to be acutely pathogenic in humans because many adenovirus based vaccines have been inoculated in human without any serious side effects. Limitations: The major limitation of the adenoviral vectors are (1) lack of sustained expression, because the viral DNA does not integrate; (2) antigenicity against viral proteins, both humoral and cytotoxic T-lymphocytes (CTL); and (3) possible toxicity at high doses.
3. Adeno-Associated Viral Vectors
One alternative vector for stable gene expression is the adeno-associated virus (AAV) vector.17 The AAV, a defective human parvovirus, can infect various types of mammalian cells. None of the tested primary cultures and established cell lines is resistant to AAV infection, and there are some preliminary data suggesting that AAV can integrate into non-dividing cells. AAV depends on the co-infection of a helper-virus, usually an adenovirus, for efficient replication. Without a helper-virus, AAV establishes latency in vivo and in vitro by integrating its genome, mostly into a single locus on chromosome 19. Although about 80% of adult population is sero-positive to AAV exposure, AAV has not been found to be associated with any diseases. The AAV genome contains a single-stranded DNA of 4675 bases. It has two large open reading frames for a replication (rep) gene and a capsid gene.17 The Rep proteins encoded by the rep gene are essential for AAV DNA replication and gene regulation. They also inhibit gene expression from heterologous promoters and cellular transformation by neomycin-resistant gene or oncogenes. There are 145 bases inverted terminal repeat sequences (ITRs) at both ends of the AAV genome, which form T-shaped hairpins serving as viral origins of DNA replication. Th...
Table of contents
- Cover
- Half Title
- Title Page
- Copyright Page
- Table of Contents
- Chapter 1 Overview
- Chapter 2 Human Hematopoietic Cells for Gene Therapy
- Chapter 3 Somatic Gene Therapy for Severe Combined Immune Deficiency (SCID)
- Chapter 4 Fibroblast Cell Biology and Gene Therapy
- Chapter 5 Hepatic Gene Therapy
- Chapter 6 Epidermal Keratinocytes: Opportunities and Applications in Somatic Cell Gene Therapy
- Chapter 7 Endothelial Cells: Endothelium as a Target Organ for Somatic Gene Therapy
- Chapter 8 Gene Therapy by Myoblast-Mediated Gene Transfer
- Chapter 9 Gene Delivery to Neurons of the Adult Mammalian Nervous System Using Herpes and Adenovirus Vectors
- Chapter 10 Implantation of Genetically Modified Cells in the Brain
- Chapter 11 Direct Gene Transfer In Vivo
- Chapter 12 Nonautologous Somatic Gene Therapy
- Chapter 13 Current Gene Marking and Gene Therapy Protocols for Human Bone Marrow Transplantation
- Chapter 14 Gene Therapy for Brain Tumors
- Chapter 15 Gene Therapy for Adult Cancers: Advances in Immunologic Approaches Using Cytokines
- Index