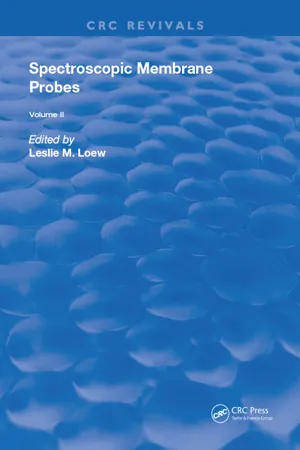
This is a test
- 228 pages
- English
- ePUB (mobile friendly)
- Available on iOS & Android
eBook - ePub
Book details
Book preview
Table of contents
Citations
About This Book
The optical spectra of molecular membrane probes can be interpreted terms of the structure, dynamics, and the physiological state of the membrane. The general picture we have of membranes and of the properties of the proteins imbedded in them, has, arguably, emerged directly from probe studies over the last 20 years. This work is designed to make these techniques accessible to a broad audience of cell biologist. The techniques discussed revolve primarily around the fluorescence of membrane probes, but applications of light absorption and Raman scattering are included. In addition to reviews of the major applications, most chapters include information on required apparatus, experimental design, data analysis, and potential pitfalls.
Frequently asked questions
At the moment all of our mobile-responsive ePub books are available to download via the app. Most of our PDFs are also available to download and we're working on making the final remaining ones downloadable now. Learn more here.
Both plans give you full access to the library and all of Perlego’s features. The only differences are the price and subscription period: With the annual plan you’ll save around 30% compared to 12 months on the monthly plan.
We are an online textbook subscription service, where you can get access to an entire online library for less than the price of a single book per month. With over 1 million books across 1000+ topics, we’ve got you covered! Learn more here.
Look out for the read-aloud symbol on your next book to see if you can listen to it. The read-aloud tool reads text aloud for you, highlighting the text as it is being read. You can pause it, speed it up and slow it down. Learn more here.
Yes, you can access Spectroscopic Membrane Probes by Leslie M. Loew in PDF and/or ePUB format, as well as other popular books in Medicine & Biochemistry in Medicine. We have over one million books available in our catalogue for you to explore.
Information
Chapter 15
POTENTIAL-SENSITIVE MOLECULAR PROBES IN ENERGY-TRANSDUCING ORGANELLES
TABLE OF CONTENTS
I. | Introduction |
II. | Energy-Transduction Models |
III. | Mitochondria and Related Preparations |
IV. | Photosynthetic Systems |
V. | Sarcoplasmic Reticulum |
VI. | Miscellaneous Preparations |
VII. | Summary Remarks |
VIII. | Practical Considerations in the Selection of Optical Probes |
Acknowledgments | |
Abbreviations Used | |
References |
I. INTRODUCTION
This chapter deals with the application of potential-sensitive optical probes to the broadly defined discipline of bioenergetics. Although in principle any system containing an ATPase can be regarded as falling into the latter category, the material contained herein is largely confined to the application of probes to energy transducing organelles. The behavior of optical molecular probes in model systems and in organ or tissue-level studies is cited only when the model system studies directly bear on the behavior of the probes in these organelles or in the latter work when the optical signals appear to be primarily due to such organelles. The use of optical indicators in model systems and in organ or tissue-level studies is covered elsewhere in this work. Whole-cell probe applications are covered by Freedman and Laris in Volume 3, Chapter 16. The contents of this chapter are based on literature searches through March 1986.
Most of the references and published information are organized in the several tables. References have been grouped into the following categories: mitochondria and related preparations, Table 1; photosynthetic systems, Table 2; sarcoplasmic reticulum, Table 3; and several miscellaneous studies, Table 4. In each table, the author(s), probe(s) employed, and a reference number are provided. The body of the text contains a discussion of several current issues regarding the application of potential-sensitive molecular probes: the specificity or lack thereof of the dye to membrane potential(s), the mechanisms by which the probes respond to charge separation, the nature of the charge separation to which the probes are sensitive, calibration procedures and attendant problems, and the kinetic competence of selected probes to follow the charge-separation events in membranes. Although an effort has been made to provide a comprehensive listing of papers involving probe usage, those studies in which the behavior of the probe in the membrane system of interest is critically examined have been given priority in the accompanying text. The application of extrinsic probes to a variety of biological preparations, including energy-transducing membranes, has been considered in reviews by Waggoner,1 Cohen and Salzberg,2 Bashford and Smith,3 and Bashford.4 Early application of a number of oxonol probes in energy-transducing membrane systems has been described in monograph articles by Chance et al.,5 Bashford et al.,6 and Smith et al.7 The utility of a number of commonly employed experimental techniques for measuring membrane potentials in mitochondria, including molecular probes, has been reviewed by Tedeschi.8
The probes are usually charged and often consist of fused ring structures, such as those characteristic of ANS, or the berberine series, or fall into the polyene group in which two ring structures of varying complexity are connected by a conjugated carbon chain. In the latter group, the wavelength of maximum absorption is a function of the length of the conjugated chain9 which can be varied to obtain dye derivatives, the optical absorption and emission spectra of which do not significantly overlap those of intrinsic membrane pigments. With the exception of carotenoids such as β-carotene and certain intrinsic energy-dependent probes such as the cytochrome c oxidase,10 these probes must be added to the system of interest in a carefully controlled fashion in order to obtain maximum energy-dependent optical signals with minimal effects on membrane viability.
II. ENERGY-TRANSDUCTION MODELS
Because the interpretation of molecular probe optical signals in response to light or substrate activation of energy-transducing preparations in terms of mechanistic and kinetic schemes involves, to some extent, the chosen model for the energy-transduction process, and, conversely, the behavior of the probe signals under energy-transducing conditions can provide insight into the validity of such models, this section presents a brief summary of several current proposals concerning energy transduction that are applicable to the areas covered in this chapter. The most commonly accepted model for energy transduction in mitochondria and preparations derived therefrom, as well as photosynthetic systems and a host of single-cell organisms is the chemiosmotic theory of Mitchell,11–13 in its original form or as modified by the introduction of Q cycles14 and variable H+/∂e- stoichiometrics. This theory is based on the presence of a transmembrane electrical potential difference ΔΨ and a pH-gradient ΔpH related according to Equation 1 generated by vectorial electron transport and the extrusion of protons to the bathing medium or proton uptake by the thylakoid space resulting in the mitochondrial matrix or the latter space of chloroplasts, respectively, being at a negative or positive potential relative to that of the external phase:
(1) |
Based on recently obtained data on the lateral diffusion of enzymes in energy-transducing membranes, Boyer15 and Slater et al.16 have suggested a direct interaction of energized redox enzymes with the ATP synthase through a transient, i.e., collisional, process in which energy is transferred to the ATPase, driving a conformational change in the latter complex that results in the spontaneous synthesis of ATP from bound ADP and Pi and the release of previously formed ATP from the tight binding site. In the model of Slater et al., the energized redox enzyme, the ubiquinol cytochrome c oxidoreductase in mitochondria, can either relax to the unergized enzyme form acting as a proton pump in the relaxation process that produces a ΔμH or drive a conformational change in the ATP synthase that may then either directly synthesize ATP as described above or function as a proton pump producing ΔμH.
More recently, however, the role of surface potentials in mitochondrial oxidative phosphorylation has received considerable attention. Malpress17 has proposed that electron transport results in the formation of fixed negative charges on or near the mitochondrial inner membrane surface. The resulting surface potential controls the concentration of ions in the diffuse double layer; the electrochemical gradient or proton motive force in this model is related to the surface electrostatic potential ΔΨH by a factor f which is a function of the prevailing experimental conditions and substrates used in oxidative phosphorylation:
(2) |
ΔμH is derived from proton movements into the bulk phase from the double-layer region which are the net effect of all competing ion movements which affect the proton concentration level in the double layer. The latter sensitivity has been suggested as an explanation for certain observations that are not in accord with the chemiosmotic model, such as the dependence of the ratio of the phosphorylation potential to the electrochemical gradient ΔμH on the value of the electrochemical gradient itself. Woelders et al.18 have, however, presented evidence that such dependence is due to an experimental artifact; when the later artifact is eliminated ΔGp:ΔμH is constant over a twofold range of ΔμH. Kell19 has proposed a somewhat similar model in which the existence of a transmembrane potential that exceeds the potetial difference between the matrix and bulk aqueous phases is suggested. Most of the functional proton translocation is supposed to occur at the surface of the mitochondrial membrane in fixed channels which contain the ATPase at a potential different from that of the bulk phase. Proposed mechanisms include a Grotthus transfer of protons between adsorbed water molecules. Like the Malpress model, the electrodic model of Kell envisions the formation of surface charges presumably due to changes in ionization, pK, or conformation of proton carriers being linked to electron transport. Van Walraven et al.20 have suggested that the effective charge gradient is either within the bilayer dielectric or across the diffuse double-layer region with the potential difference between the two bulk phases serving as a “buffer” to drive ion translocation, or to smooth out fluctuations in ATP synthesis or ...
Table of contents
- Cover
- Title Page
- Copyright Page
- Dedication
- Chapter 9 Fluorescence Correlation Spectroscopy and Photobleaching Recovery: Measurement of Transport and Chemical Kinetics
- Chapter 10 Cell Surface Clustering and Mobility of the Liganded LDL Receptor Measured by Digital Video Fluorescence Microscopy
- Chapter 11 Total Internal Reflection Fluorescence: Theory and Applications at Biosurfaces
- Chapter 12 Near-Field Imaging of Fluorescence
- Chapter 13 Fluorescent Probes of Anion Transport Inhibition in the Human Red Cell Membrane
- Chapter 14 How to Choose a Potentiometric Membrane Probe
- Chapter 15 Potential-Sensitive Molecular Probes in Energy-Transducing Organelles
- Index