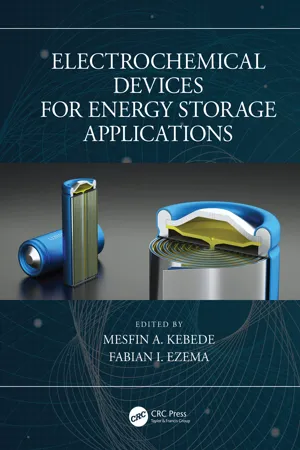
eBook - ePub
Electrochemical Devices for Energy Storage Applications
This is a test
- 280 pages
- English
- ePUB (mobile friendly)
- Available on iOS & Android
eBook - ePub
Electrochemical Devices for Energy Storage Applications
Book details
Book preview
Table of contents
Citations
About This Book
This book explores a wide range of energy storage devices, such as a lithium ion battery, sodium ion battery, magnesium ion battery and supercapacitors. Providing a comprehensive review of the current field, it also discusses the history of these technologies and introduces next-generation rechargeable batteries and supercapacitors. This book will serve as a valuable reference for researchers working with energy storage technologies across the fields of physics, chemistry, and engineering.
Features:
• Edited by established authorities in the field, with chapter contributions from subject area specialists
• Provides a comprehensive review of field
• Up to date with the latest developments and research
Frequently asked questions
At the moment all of our mobile-responsive ePub books are available to download via the app. Most of our PDFs are also available to download and we're working on making the final remaining ones downloadable now. Learn more here.
Both plans give you full access to the library and all of Perlego’s features. The only differences are the price and subscription period: With the annual plan you’ll save around 30% compared to 12 months on the monthly plan.
We are an online textbook subscription service, where you can get access to an entire online library for less than the price of a single book per month. With over 1 million books across 1000+ topics, we’ve got you covered! Learn more here.
Look out for the read-aloud symbol on your next book to see if you can listen to it. The read-aloud tool reads text aloud for you, highlighting the text as it is being read. You can pause it, speed it up and slow it down. Learn more here.
Yes, you can access Electrochemical Devices for Energy Storage Applications by Mesfin A. Kebede, Fabian I. Ezema, Mesfin A. Kebede, Fabian I. Ezema in PDF and/or ePUB format, as well as other popular books in Physical Sciences & Physics. We have over one million books available in our catalogue for you to explore.
1
Layered, Spinel, Olivine, and Silicate as Cathode Materials for Lithium-Ion Battery
Mesfin A. Kebede, Nithyadharseni Palaniyandy, and Lehlohonolo F. Koao
CONTENTS
1.1 Introduction
1.1.1 The Working Principles of LIB
1.2 Layered LiCoO2, LiMnO2, LiNiO2, LiCo0.33Mn0.33Ni0.33O2, xLi2MnO3(1–x)LiMO2 (M = Transition Metals), Li2MnO2
1.2.1 Layered LiCoO2, LiMnO2, LiNiO2, LiCo0.33Mn0.33Ni0.33O2
1.2.1.1 LiCoO2
1.2.1.2 LiMnO2
1.2.1.3 LiNiO2
1.2.1.4 LiCo0.33Mn0.33Ni0.33O2
1.2.2 Lithium- and Manganese-Rich Layered Structure Materials
1.2.3 Nickel-Rich, LiNixCoyMnzO2 (x > 0.6)
1.3 Spinel LiMn2O4 and LiMn1.5Ni0.5O4 (LMNO)
1.3.1 LiMn2O4
1.3.2 LiMn1.5Ni0.5O4
1.4 Olivine Cathodes
1.4.1 LiFePO4
1.4.2 LiMnPO4
1.4.3 LiNiPO4
1.5 Silicate Cathode: Li2MSiO4, M = Fe and Mn
1.5.1 Li2FeSiO4
1.5.2 Li2MnSiO4
1.6 Conclusions
References
1.1 Introduction
Rechargeable lithium-ion battery (LIB) not only has played a substantial role of powering portable electronic gadgets throughout the past decades, but also contributed in revolutionizing electronics technology advancement since its introduction by Sony in 1990. LIB is so attractive owing to the fact that it could provide a high-energy density with small size, has longer cycle life, has no memory effect, etc. as compared to its predecessor rechargeable battery chemistries (lead acid, Ni–Cd, Ni MH, etc.), as shown in Figure 1.1 (Tarascon and Armand 2011, 171–79).
Along with continuing market expansion and increasing demand, apart from the famous dominance on electronics, LIB is being implemented in various areas of technology such as to power electric bicycles and electric vehicles (EVs). Moreover, the use and significance of LIB keeps on gaining tremendous momentum than ever before, as its use becomes multifunctional and very diverse along with the rise of the 4th Industrial Revolution (4IR) characterised by hyper-connectivity which encompasses artificial intelligence, Internet of Things, big data, etc. The other breakthrough is wearable devices such as smartwatches, smart eyeglasses, fitness-tracking bands, etc. All these aforementioned technology advancements require and consume more power with less volume acquiring. For this, LIB is still the best energy source option. The other recent research hotspot is the flexible lithium ion batteries, by improving designs and functions.
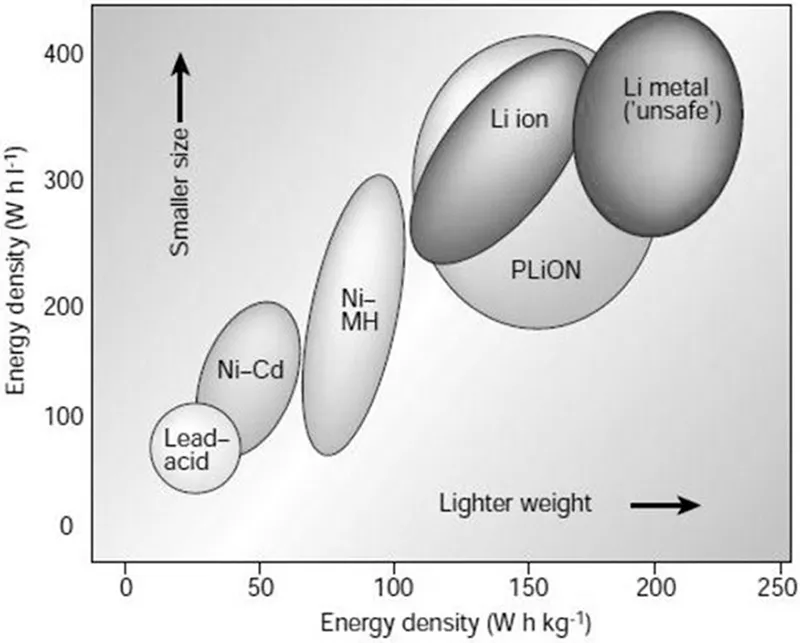
1.1.1 The Working Principles of LIB
A LIB is an electrochemical cell (transducer) which functions as the energy storage device by converting electric energy into electrochemical energy and vice versa. The schematic diagram in Figure 1.2 represents the very basic fundamental working principles of LIB. LIB basically consists of three main components that are cathode (positive electrode), the anode (negative electrode), and electrolyte. Normally, between the cathode and anode, there will be a separator which mechanically separates the positive and negative electrodes to prevent short circuiting, which could lead to explosion (Zhang 2007, 351–64; Hao et al. 2013, 11–16; Liu et al. 2018, 265–75). This separator is designed in such a way with the appropriate size of porosity to allow maximum ionic conductivity of the lithium ion-containing electrolyte (Weber et al. 2014, 66–81).
Typically, rechargeable batteries are characterised and evaluated by their energy density (Wh kg−1), specific energy (Wh L−1), specific power (W kg−1), cycle life, efficiency, and cost. This in turn is determined by the performance of the components that make up the battery cell: negative and positive electrodes, as well as separator and electrolyte. Importantly, the capacity and voltage of the electrodes determine the energy density and specific energy of the cell, as seen in equations (1.1) and (1.2).
(1.1) |
(1.2) |
Interestingly, though the cathode electrodes usually provide a low discharge capacity as compared to the anode, they actually dictate the overall parameters of the cell, such as the voltage, the capacity, and energy density. Thus, the developments of high-energy and high-power density cathode materials become extremely crucial and have received much attention in recent times.
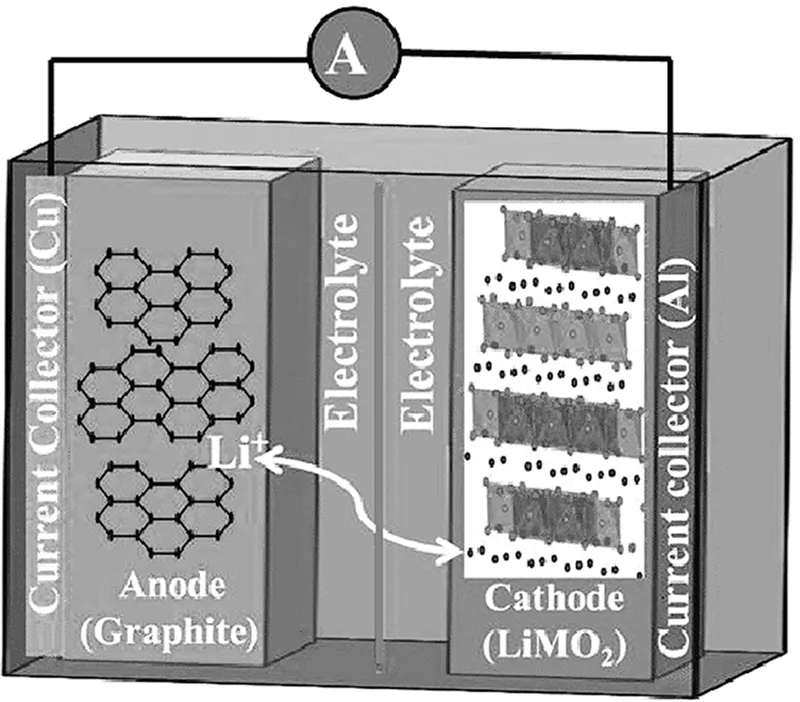
There are various types of cathode materials being researched for LIB applications. However, the scope of this chapter only focuses on the most famous and commercialized categories of cathodes of lithium ion batteries, namely, layered LiMO2 (M=Co, Mn, Ni), spinel LiMn2O4, olivine LiFePO4,and silicate Li2MSiO4 structures which are also commercially utilized in the global market. In this chapter, we discuss them in detail as topics of the chapter.
1.2 Layered LiCoO2, LiMnO2, LiNiO2, LiCo0.33Mn0.33Ni0.33O2, xLi2MnO3(1 − x)LiMO2 (M = Transition Metals), Li2MnO2
The most notable cathode for LIB, layered LiCoO2 (LCO) is isostructural to the α-NaFeO2-type structure that has rhombohedral structure and has trigonal symmetry with the R−3m space group (No. 166), which has lithium and cobalt ions located in octahedral 3a and 3b sites of cubic close-packed (ccp) of the oxygen stacks, respectively. There are three slabs of edge-sharing CoO6 octahedra separated by interstitial layers of Li in the unit cell of the layered LiCoO2 as shown in Figure 1.3. As lithium ions intercalate/deintercalate during charging and discharging, the crystal structure dynamics change. When LiCoO2 is in a fully lithiated state, it remains in layered structures with hexagonal unit cells. During removal of Li from the layered crystal lattice, as charging implemented the nonstoichiometric Li1−xCoO2 compounds to form, and, correspondingly, the oxidation of Co3+ changes to Co4+ as the charge compensation process takes place.
Currently, the large family of lithium intercalation cathode materials (LiCoO2, LiMnO2, LiNiO2, LiCo0.33Mn0.33Ni0.33O2, etc.), in relation to a layered rhombohedral structure (R−3m symmetry), are being introduced and are believed to be the next-generation cathode materials, as they have potential to provide relatively high and reasonable capacity and high voltage. When considered for practical usage in electric vehicles and portable power devices, each end-member comes with its own advantages and shortcomings. For instance, the manganese-based LiMnO2 showed promise due to its lower cost, lower toxicity, higher safety, and comparable high theoretical capacity (∼285 mA h g−1). Nevertheless, the challenge of this layered cathode material is found to be metastable thermodynamically, converting to the cubic spinel structure LiMn2O4 (Fd3m symmetry) during electrochemical deintercalation/intercalation. The LiMnO2 does not easily form a layered R–3m structure (Armstrong and Bruce 1996, 499), instead, it forms the orthorhombic phase structure that causes low rate capability and capacity (Amatucci et al. 1997, 11–25).
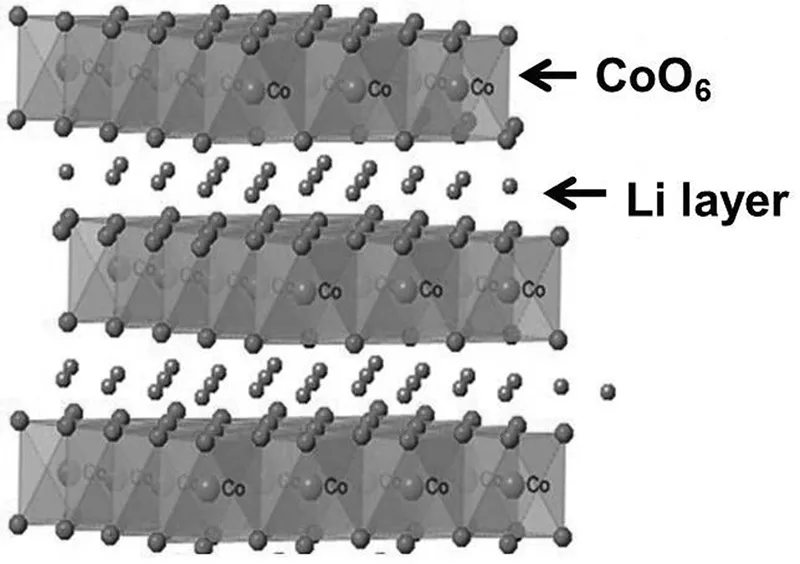
The other individual end-member is LiNiO2 (LNO). Dyer et al. initially synthesised LNO by bubbling oxygen through lithium hydroxide at about 800°C in 1954 (Dyer, Borie Jr, and Smith 1954, 1499–503). In the late 1980s, Dahn et al. extensively studied LNO to use it as an alternative to LCO. LNO is isostructural to LCO (R(−)3m), and thus structurally speaking, it should easily allow for lithium intercalation. The benefit of LNO is that nickel is more abundant than cobalt and would make for a cheaper alternative. Some of the drawbacks of LNO are the synthesis of LNO is very difficult due to the instability of the trivalent nickel at elevated temperature and strongly dependant on appropriate conditions (depends on precursors, oxygen atmosphere, annealing temperature). The other drawback is LiNiO2 suffers Ni2+ mixing in the Li+ layer that provides d...
Table of contents
- Cover
- Half Title
- Title Page
- Copyright Page
- Table of Contents
- Preface
- Editors
- Contributors
- 1. Layered, Spinel, Olivine, and Silicate as Cathode Materials for Lithium-Ion Battery
- 2. Metal Oxide-Based Anode Materials for Lithium-Ion Battery
- 3. Sodium-Ion Battery Anode Materials and Its Future Prospects and Challenges
- 4. Cathode Materials for Sodium-Ion-Based Energy Storage Batteries
- 5. Magnesium Battery
- 6. Graphene-Based Electrode Materials for Supercapacitor Applications
- 7. Transition Metal Oxide-Based Nanomaterials for High Energy and Power Density Supercapacitor
- 8. The Role of Modelling and Simulation in the Achievement of Next-Generation Electrochemical Capacitors
- 9. Cerium Oxide: Synthesis, Structural, Morphology, and Applications in Electrochemical Energy Devices
- 10. Multifunctional Energy Storage: Piezoelectric Self-charging Cell
- 11. The Contributions of Electrolytes in Achieving the Performance Index of Next-Generation Electrochemical Capacitors (ECs)
- Index