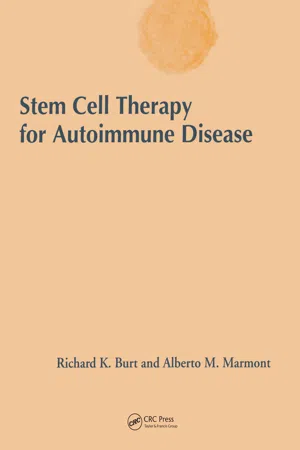
- 512 pages
- English
- ePUB (mobile friendly)
- Available on iOS & Android
Stem Cell Therapy for Autoimmune Disease
About This Book
Stem cell transplantation may be complicated by treatment-related mortality and like the immune system that it regenerates has equal potential to either create and preserve or destroy. The dual nature that defines stem cells is differentiation that ultimately leads to death and self-renewal, which leads to immortality. What types of stem cells are there? How are they collected? What are their attributes and characteristics? This textbook devotes many chapters to familiarize the reader with the basic science, clinical aspects, and new questions being raised in the field of stem cell biology. Blood stem cells for tolerance and tissue regeneration are a rapidly developing research and clinical field that is being applied to autoimmune diseases. In clinical trials, autologous hematopoietic (blood) stem cells are being used to reduce the cytopenic interval following intense immune suppressive transplant regimens. While as yet not delineated, some possible mechanisms and pathways leading to tolerance after hematopoietic stem cell transplantation are suggested in these chapters. Tissue regeneration from blood stem cells is also suggested by animal experiments on stem cell plasticity or metamoirosis (i.e., change in fate) as described within this textbook. Ongoing early clinical trials on tissue regeneration from blood stem cells are described in the chapter on stem cell therapy for cardiac and peripheral vascular disease. Whether autologous hematopoietic stem cells, through the process of mobilization and reinfusion, may be manipulated to contribute to tissue repair in autoimmune diseases is a future area for translational research.
Frequently asked questions
Information
CHAPTER 1
When is a Stem Cell Really a Stem Cell?
Introduction
Embryonic Stem Cells: A Timeline

Mouse to Man
Adult Stem Cells
Hematopoietic Stem Cells: Paradigms for Stem Cell Biology
Table of contents
- Cover
- Half Title
- Title Page
- Copyright Page
- Dedication Page
- Table of Contents
- Editors
- Contributor
- Frontispiece
- Preface
- 1. When is a Stem Cell Really a Stem Cell?
- 2. Embryonic Stem Cells: Unique Potential to Treat Autoimmune Diseases
- 3. Neural Stem Cells and Oligodendrocyte Progenitors in the Central Nervous System
- 4. Turning Blood into Liver
- 5. Adipose Tissue-Derived Adult Stem Cells: Potential for Cell Therapy
- 6. Hematopoietic Stem Cell Biology: Relevance to Autoimmunity
- 7. Properties and Therapeutic Potentials of Adult Stem Cells from Bone Marrow Stroma (MSCs)
- 8. Regeneration of Cardiomyocytes from Bone Marrow Stem Cells and Application to Cell Transplantation Therapy
- 9. Clinical Trials of Hematopoietic Stem Cells for Cardiac and Peripheral Vascular Diseases
- 10. The Stem Cell Continuum: A Plastic Plasticity
- 11. Adult Stem Cell Plasticity
- 12. Collection and Expansion of Stem Cells
- 13. The Extracellular Matrix as a Substrate for Stem Cell Growth and Development and Tissue Repair
- 14. Gene Transfer into Human Hematopoietic Stem Cells: Problems and Perspectives
- 15. The Etiopathogenesis of Autoimmunity
- 16. Overview of Immune Tolerance Strategies
- 17. Death Receptor-Mediated Apoptosis and Lymphocyte Homeostasis
- 18. Shifting Paradigms in Peripheral Tolerance
- 19. Dendritic Cells Control the Balance between Tolerance and Autoimmunity
- 20. CD4+ ΀ Regulatory Cells and Modulation of Undesired Immune Responses
- 21. Major Histocompatibility Complex and Autoimmune Disease
- 22. Analyzing Complex Polygenic Traits: The Role of Non-HLA Genes in the Susceptibility to Autoimmune Disorders
- 23. Drug-Induced Autoimmunity
- 24. Evidence for a Role of Infections in the Activation of Autoreactive ΀ Cells and the Pathogenesis of Autoimmunity
- 25. Molecular Analysis of Immunity
- 26. Immune Reconstitution after Hematopoietic Stem Cell Transplantation
- 27. Historical Perspective and Rationale of HSCT for Autoimmune Diseases
- 28. High-Dose Immune Suppression without Hematopoietic Stem Cells for Autoimmune Diseases
- 29. Autologous Stem Cell Transplantation in Animal Models of Autoimmune Diseases
- 30. Allogeneic Hemopoietic Stem Cell Transplantation in Animal Models of Autoimmune Disease
- 31. Mobilization and Conditioning Regimens in Stem Cell Transplant for Autoimmune Diseases
- 32. Infection in the Hematopoeitic Stem Cell Transplant Recipient with Autoimmune Disease
- 33. Immunological Aspects of Multiple Sclerosis with Emphasis on the Potential Use of Autologous Hemopoietic Stem Cell Transplantation
- 34. Axonal Injury and Disease Progression in Multiple Sclerosis
- 35. Monitoring Disease Activity in Multiple Sclerosis
- 36. Intense Immunosuppression Followed by Autologous Stem Cell Transplantation in Severe Multiple Sclerosis Cases: MRI and Clinical Data
- 37. Hematopoietic Stem Cell Transplantation for Multiple Sclerosis: Finding Equipoise
- 38. Molecular and Cellular Pathogenesis of Systemic Lupus Erythematosus
- 39. Definition, Classification, Activity and Damage Indices in Systemic Lupus Erythematosus
- 40. Lupus Nephritis
- 41. Hematopoietic Stem Cell Transplantation for Systemic Lupus Erythematosus
- 42. Treatment of Rheumatoid Arthritis
- 43. Haemopoietic Stem Cell Transplantation for Rheumatoid ArthritisâWorld Experience and Future Trials
- 44. Autologous Stem Cell Transplantation for Refractory Juvenile Idiopathic Arthritis (JIA)
- 45. Immunology of Scleroderma
- 46. Hematopoietic Stem Cell Transplantation for Systemic Sclerosis
- 47. High-Dose Immunosuppressive Chemotherapy with Autologous Stem Cell Support for Chronic Autoimmune Thrombocytopenia
- 48. High-Dose Chemotherapy with Haematopoietic Stem Cell Transplantation in Primary Systemic Vasculitis, Behcetâs Disease and Sjogrenâs Syndrome
- 49. Hematopoietic Stem Cell Transplantation in the Treatment of Chronic Inflammatory Demyelinating Polyradiculoneuropathy
- 50. Hematopoietic Stem Cell Therapy for Patients with Refractory Myasthenia Gravis
- 51. Hematopoietic Stem Cell Transplantation in Patients with Autoimmune Bullous Skin Disorders
- 52. Idiopathic Inflammatory Myositis
- 53. Hematopoietic Stem Cell Transplantation as Treatment for Type 1 Diabetes
- 54. Autologous Hematopoietic Stem Cell Transplantation for Crohnâs Disease
- 55. Bronchial Asthma and Idiopathic Pulmonary Fibrosis as Potential Targets for Hematopoietic Stem Cell Transplantation
- 56. Autologous Stem Cell Transplantation in Relapsing Polychondritis
- 57. Allogeneic Hematopoietic Stem Cell Transplantation for Autoimmune Diseases
- Index