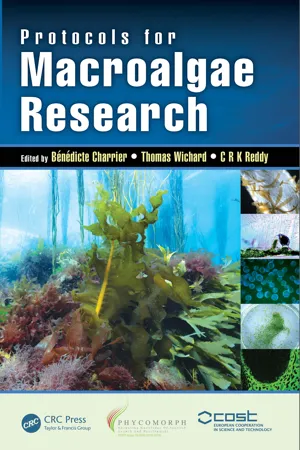
eBook - ePub
Protocols for Macroalgae Research
This is a test
- 496 pages
- English
- ePUB (mobile friendly)
- Available on iOS & Android
eBook - ePub
Protocols for Macroalgae Research
Book details
Book preview
Table of contents
Citations
About This Book
This book presents a wide range of tested and proven protocols relevant to a number of fields within biotechnology used in laboratory experiments in everyday phycological (seaweed) research. A major focus will be on bioenergy related aspects of this emerging technology. These protocols will be written in a clear and concise manner using simple language permitting even nonspecialist to adequately understand the significance of this research. It will also contain all necesssary notes and guidelines for successful execution of these experiments.
Frequently asked questions
At the moment all of our mobile-responsive ePub books are available to download via the app. Most of our PDFs are also available to download and we're working on making the final remaining ones downloadable now. Learn more here.
Both plans give you full access to the library and all of Perlego’s features. The only differences are the price and subscription period: With the annual plan you’ll save around 30% compared to 12 months on the monthly plan.
We are an online textbook subscription service, where you can get access to an entire online library for less than the price of a single book per month. With over 1 million books across 1000+ topics, we’ve got you covered! Learn more here.
Look out for the read-aloud symbol on your next book to see if you can listen to it. The read-aloud tool reads text aloud for you, highlighting the text as it is being read. You can pause it, speed it up and slow it down. Learn more here.
Yes, you can access Protocols for Macroalgae Research by Bénédicte Charrier, Thomas Wichard, C R K Reddy, Bénédicte Charrier, Thomas Wichard, C R K Reddy in PDF and/or ePUB format, as well as other popular books in Biological Sciences & Marine Biology. We have over one million books available in our catalogue for you to explore.
Information
section two
Chemical composition
chapter ten
Biochar production from seaweeds
Contents
10.1 Introduction
10.2 State of the art
10.3 Materials
10.3.1 Extraction and pre-drying of seaweed biomass
10.3.2 Sample preparation by pyrolysis
10.3.3 Biochar generation by pyrolysis
10.3.4 Storage of biochar samples
10.4 Experimental procedures
10.4.1 Extraction and pre-drying of seaweed biomass (the case of Macrocystis pyrifera)
10.4.2 Sample preparation for pyrolysis
10.4.3 Biochar generation by pyrolysis
10.4.4 Storage of biochar samples
10.5 Notes
Acknowledgments
References
10.1 Introduction
Biochar is a carbon-rich solid derived from thermally stabilized organic material, meaning that this product can be stored and applied to soils for improving its quality. Biochar differs from other solid products derived from thermochemical conversion, such as charcoal or active carbon, in that long-term carbon storage is an objective, than the generation of raw material for processing industries or fuels (Mašek et al. 2013). In technical terms, biochar is produced through the thermal decomposition of organic material under oxygen (O2)-limited conditions and at relatively low temperatures (<900°C) (Lehmann and Joseph 2009; Cha et al. 2016). Through a process termed pyrolysis, biochar production results in subproducts such as gases (e.g., synthesis gas) and liquids (e.g., tars and oils) (Bridgwater and Peacocke 2000; Cha et al. 2016). At lower temperatures (<550°C), pyrolysis tends to produce more biochar, whereas at higher temperatures, more synthesis gas is generated.
In recent years, biochar has been a subject of important scientific and commercial interest because of its role in carbon sequestration, ability to improve nutrient uptake efficiency in soils, and capacity to adsorb pollutants from diverse matrices. In relation to improving soils, several studies demonstrate that biochar incorporation can improve (1) nutrient retention; (2) cationic interchange capacity; (3) chemical, physical, and biological soil properties; and (4) plant health and growth, in addition to reducing nitrogen loss in fertilized soils through volatilization and lixiviation (Jassal et al. 2015; Mandal et al. 2016). Adding biochar to soils also reduces the emission of greenhouse gases such as CH4 and N2O (Qian et al. 2015). For example, Case et al. (2015) reported that biochar suppresses the accumulated production of N2O by 91% in near-saturated fertilized soils. Furthermore, denitrification is reduced by 37%, which represents between 85% and 95% of N2O emissions in soil.
The use of low-cost raw materials is of vital importance for future biochar applications. Consequently, biochar production using seaweeds has garnered widespread attention as a promising alternative. The low cost of this raw material is because of macroalgae abundance and easy collection from natural fields or detachments, and from seaweed farms. Research associated with the use of biochar originating from macroalgae primarily focuses on the use of this raw material as a low-cost adsorbent of pollutants, mainly in the removal of metals (Cho et al. 2013; Park et al. 2016) and phosphate (Jung et al. 2015a, b: 2016a, b) from aquatic systems.
Scientific studies assessing the use of seaweed-derived biochar in soils are fewer and more recent. For example, Bird et al. (2011) studied the potential of different types of seaweeds on biochar generation and, subsequently, applications in carbon sequestration, as a soil amender, and as a fertilizer. Roberts et al. (2015a, b) used biochar generated from freshwater macroalgae (Oedogonium) cultivated in a wastewater of a coal-fired power station to enhance the rehabilitation of soils close to a coal mine. Cole et al. (2017) used the same species to recover nitrogen (N) and phosphorus (P) from municipal wastewater and then used the cultivated algae to produce biochar as a soil amendment. As such, algal biomass use is now considered as a new biotechnological alternative (Lee and Woo 2014) from an environmental point of view.
10.2 State of the art
Biochar can be obtained from different biomass feedstock, including wood residue, crop residue, grass, manure, sludge and municipal organic waste, and seaweeds, among others. Successful biochar use depends on the properties of the originating biomass and operational conditions, including residency time, temperature, heating rate, reactor type, and so on, among others (Rajapaksha et al. 2016). As such, biochar produced from seaweeds has a low carbon content compared with biochar from lingocellulosic materials and, conversely, higher concentrations of macronutrients (i.e., N and P) and interchangeable trace elements (i.e., Ca, Mg, K, and Mo), which are essential for plant growth (Bird et al. 2011; Roberts et al. 2015a, b). Nevertheless, the final composition of biochar, and the physicochemical properties varies depending on the seaweed used for production (Table 10.1). Bird et al. (2012) compared biochar derived from freshwater (FW) and saltwater (SW) macroalgae with lignocellulosic biochar (commercial charcoal). Lignocellulosic biochar was similar to the algal biochar in terms of pH, CEC, and surface area characteristic, but has considerable higher carbon content (72%) compared with FW biochar (11.6%) and SW biochar (17.4%). In turn, algal biochar presents higher ash content and considerable highest nutrient (N, P, and K) contents than lignocellulosic biochar. This final trait permits using algal biochar as a soil amender (Bird et al. 2011, 2012).
10.3 Materials
10.3.1 Extraction and pre-drying of seaweed biomass
• Plastic bags.
• Raschel mesh.
• Tap water.
• Distilled water.
• Hemp or cotton rope, 10–20 mm in diameter.
• Ice pack.
• Knife.
• Bag sealer.
10.3.2 Sample preparation by pyrolysis
• Drying oven.
• Plastic or stainless-steel baskets.
• Sealable bags.
• Stainless-steel mill.
• No. 18 (1.0 mm) and N° 3.5 (5.7 mm) sieve.
• Bag sealer.
• Semi-analytical balance.
Table 10.1 Main physicochemical characteristics of biochar generated from different biomass sources and different pyrolysis conditions
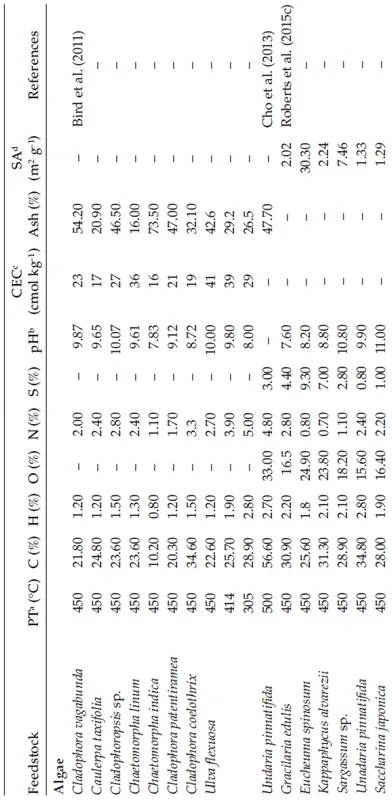

a PT, Final pyrolysis temperature.
b May differ due to the method for pH measurement, see references.
c Cation exchange capacity.
d Surface area.
10.3.3 Biochar generation by pyrolysis
• Pyrolysis reactor with speed/temperature controls, supplemented with thermocouples and gas inlet/outlet with condenser.
• Pure gaseous nitrogen (N2).
10.3.4 Storage of biochar samples
• No. 35 (0.5 mm) and 18 (1.0 mm) sieve.
• Sealable bags.
• Bag sealer.
• Stainless-steel mill.
• Porcelain mortar.
10.4 Experimental procedures
10.4.1 Extraction and pre...
Table of contents
- Cover
- Half Title
- Title Page
- Copyright Page
- Table of Contents
- Preface
- Editors
- Contributors
- Section I: Cultivating and preserving seaweeds
- Section II: Chemical composition
- Section III: Cellular and molecular characterization
- Index