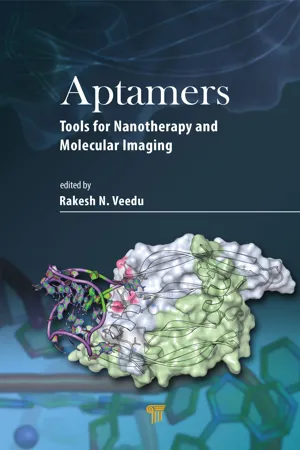
This is a test
- 386 pages
- English
- ePUB (mobile friendly)
- Available on iOS & Android
eBook - ePub
Book details
Book preview
Table of contents
Citations
About This Book
Aptamers, often termed as 'chemical antibodies, ' are an emerging class of synthetic ligands for efficient target-specific molecular recognition. The objective of this book is to highlight recent advances and potential of aptamers in various disease conditions.. This book focuses on the applications of aptamers in targeted nanotherapy, detection, and in molecular imaging in various disease conditions such as cancer, neurological diseases and infectious diseases.
Frequently asked questions
At the moment all of our mobile-responsive ePub books are available to download via the app. Most of our PDFs are also available to download and we're working on making the final remaining ones downloadable now. Learn more here.
Both plans give you full access to the library and all of Perlego’s features. The only differences are the price and subscription period: With the annual plan you’ll save around 30% compared to 12 months on the monthly plan.
We are an online textbook subscription service, where you can get access to an entire online library for less than the price of a single book per month. With over 1 million books across 1000+ topics, we’ve got you covered! Learn more here.
Look out for the read-aloud symbol on your next book to see if you can listen to it. The read-aloud tool reads text aloud for you, highlighting the text as it is being read. You can pause it, speed it up and slow it down. Learn more here.
Yes, you can access Aptamers by Rakesh N. Veedu, Rakesh N. Veedu in PDF and/or ePUB format, as well as other popular books in Médecine & Biochimie en médecine. We have over one million books available in our catalogue for you to explore.
Information
Chapter 1
History of Aptamer Development
Nasa Savory, Koichi Abe, Taiki Saito, and Kazunori Ikebukuro
Department of Biotechnology and Life Science, Tokyo University of Agriculture and Technology, 2-24-16 Naka-cho, Koganei, Tokyo 184-8588, Japan
1.1 Introduction
Aptamers are ribo- and deoxyribo-oligonucleotide ligands, first described in 1990 by Tuerk and Gold [1] and Ellington and Szostak [2]. The name “aptamer” comes from the Greek aptus, meaning “to fit,” and merus, meaning “particle.” Aptamers adopt complex three-dimensional structures capable of recognizing target molecules with high affinity and specificity comparable with those of antibodies. Over the past two decades, aptamers have been developed for a variety of target molecules such as metal ions, small molecules, peptides, proteins, microorganisms, cells, and tissues. Because aptamers are chemically produced, there is little or no batch-to-batch variation and aptamers can be easily modified with functional groups and molecular probes. Furthermore, complex aptamer structures can be reversibly denatured at high temperatures. Their structures and structural changes occurring upon target binding can be designed on the basis of Watson–Crick base pairing. Aptamers also elicit little or no immunogenicity in therapeutic applications. Owing to those characteristics, aptamers have rapidly become alternatives to antibodies [3] in various applications including but not limited to therapeutics, drug delivery, molecular imaging, clinical diagnostics, biomarker discovery, food safety, and environmental monitoring. As the first therapeutic aptamer, pegaptanib (Macugen) was approved in 2004 by the US Food and Drug Administration for the treatment of neovascular age-related macular degeneration. Some aptamers are commercially used in analytical and medical applications, and more than 10 aptamers are in clinical trials [4].
Systematic evolution of ligands by exponential enrichment (SELEX) has been the most common method for identifying aptamers since the first reports in 1990 [1, 2]. The SELEX procedure comprises repetitive cycles of sequential steps. The process is initiated by binding target molecules with a synthesized random RNA/DNA library containing more than 1014 different sequences. Oligonucleotides bound to target molecules are separated to yield a population displaying high affinity toward target molecules. The target-bound oligonucleotides are then amplified by polymerase chain reaction (PCR) to prepare a single-stranded oligonucleotide library for the next cycle of sequential steps. This selection process is usually continued for 5–20 rounds with increased selection stringency. SELEX with many methodological improvements has been used for the development of aptamers for various target molecules.
Although almost all aptamers are selected through SELEX, there are some drawbacks in the SELEX process, and aptamers selected by this method often exhibit a lack of affinity and/or specificity. For example, by-product formation in the PCR amplification step of SELEX is a typical problem in the process [5]. Separation of a binding population from the oligonucleotide library is also a critical point for obtaining aptamers. To overcome these problems and to improve the efficiency of aptamer development, many methodological improvements have been made in the SELEX process.
Post-SELEX strategies to improve and fine-tune aptamer functions have also been established. In vitro recombination and reselection from partially randomized aptamers have been shown to achieve improvement of binding properties for specific targets. Post-SELEX modification and engineering of aptamers have been attempted to improve aptamer functions. Artificial nucleotides and unnatural bases have been widely introduced to increase resistance to nucleases and pharmacokinetics of aptamers and to improve target-binding ability. In addition, in silico selection and sequence optimization have been used to optimize aptamer sequences and structures to achieve desired improvements in aptamer functions. These various post-SELEX strategies for improving aptamer function have led to the development of promising aptamers and are opening new opportunities for the application of aptamers.
In this chapter, we describe SELEX strategies with methodological improvements, well established since the first application in aptamer development. We further present a brief description of post-SELEX improvements of aptamers that enable the development of aptamers with desired binding properties and functions.
1.2 In vitro Selection
Since the first aptamer selections were reported, most aptamers have been selected by SELEX. Many researchers have also engaged in improvement of the SELEX methodology to efficiently select aptamers, as comprehensively reviewed by Darmostuk et al. [6]. SELEX consists of four steps: incubation of an oligonucleotide library with target proteins, partitioning of oligonucleotides bound to target molecules, amplification of eluted oligonucleotides by PCR, and preparation of single-stranded DNA/RNA for subsequent cycles (Fig. 1.1). The simplicity of these cycles has allowed their automation, potentially reducing the time and cost of aptamer development [7]. Among the SELEX processes, separation of bound from unbound oligonucleotides is one of the most important steps, given that efficient fractionation allows the reduction of the SELEX cycle number, shortening the development time of aptamers and saving costs. Moreover, efficient fractionation enables the reduction of PCR amplification steps, which sometimes leads to the loss of high-affinity aptamers and the amplification of unexpected oligonucleotides [8, 9].

Membranes and beads are commonly used to immobilize target proteins for fractionations. They can readily separate oligonucleotides bound to target molecules from other oligonucleotides. However, we need to consider the possibility of the enrichment of oligonucleotides binding to these supports. Capillary electrophoresis (CE) is a useful strategy for removing unbound oligonucleotides without supports [10]. Because CE-SELEX can efficiently partition oligonucleotides binding to target molecules, Berezovski proposed selection of aptamers without amplification steps, a process named non-SELEX [11]. Other researchers have also reported efficient fractionations using a microfluidic system combined with magnetic beads (reviewed in Ref. [12]) or flow cytometry combined with emulsion PCR [13].
1.2.1 Library for SELEX
Diversity of the oligonucleotide library is another important factor for identification of aptamers [14]. Because in vitro selection of aptamers is analogous to random search in a sequence–function space, the diversity of the library is representative of the search space. We can typically use 1–10 nmol of oligonucleotides as the initial library equivalent of 1014–1015 molecules, which theoretically covers the diversity of 24–26-mer randomized oligonucleotides. Although the whole sequence space cannot be searched if we use longer oligonucleotides, longer sequences are sometimes favored to be used for selection of aptamers, because a library consisting of longer sequences can contain more complex functional modules and several simple functional motifs in a sequence. However, we need to handle longer libraries carefully because they may easily form multimers via intermolecular hybridization.
The sequences flanking constant regions of a library, which hybridize with primers during PCR process in SELEX, are also important for successful aptamer selection. We need to select constant regions to amplify selected DNA efficiently with limited nonspecific amplification. In addition, we need to consider the interference of hybridization between constant and random regions, resulting in decreased diversity of functional sequence motifs. Hybridization of antisense oligonucleotides with single-stranded DNA in a library helps in reducing interference, and it is very useful for constructing truncated mutants of aptamers [15–17]. Primerless SELEX has also been reported [18]. However, many researchers still use a library harboring a primer hybridization region. Hybridization of antisense DNA to the defined region helps minimize the effect of a defined flanking region on the folding of a random region and also in truncating the defined sequence effectively [16].
Parallel-type sequencers, as represented by Illumina instruments, have caused a revolution in biology. We can sequence not only whole genomes in a day but also whole transcriptomes, including unidentified transcripts, which is impossible by microarray analysis. They have also caused a revolution in aptamer selection. In conventional SELEX, we analyze some 100–200 sequences by Sanger sequencing after cloning into an appropriate plasmid vector. To discriminate enriched sequences from others, we should perform many SELEX cycles to enrich sequences to more than 10% of the population. However, because parallel-type sequencers permit the analysis of s...
Table of contents
- Cover
- Half Title
- Title Page
- Copyright Page
- Table of Contents
- Preface
- 1 History of Aptamer Development
- 2 Thioredoxin-Based Peptide Aptamers: Development and Applications
- 3 Aptamer Selection Methodologies
- 4 Selection and Application of Catalytically Active Oligonucleotides
- 5 Stem-Cell-Specific Aptamers for Targeted Cancer Therapy
- 6 Aptamers as Therapeutic Tools in Neurological Diseases
- 7 Aptamers in Bacterial, Viral, and Parasitic Diseases
- 8 Aptamers as Tools for Targeted Drug Delivery
- 9 Aptamer–Liposome Conjugates: Current Art and Future Prospects
- 10 Aptamers in Medical Diagnosis
- 11 Molecular Imaging Utilizing Aptamer-Targeted Probes
- 12 First Therapeutic Aptamer: VEGF-Targeting Macugen
- 13 Aptamers: Scope, Limitations, and Future Prospects
- Index