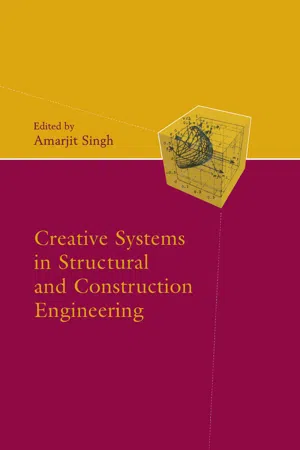
This is a test
- 1,040 pages
- English
- ePUB (mobile friendly)
- Available on iOS & Android
eBook - ePub
Creative Systems in Structural and Construction Engineering
Book details
Book preview
Table of contents
Citations
About This Book
An examination of creative systems in structural and construction engineering taken from conference proceedings. Topics covered range from construction methods, safety and quality to seismic response of structural elements and soils and pavement analysis.
Frequently asked questions
At the moment all of our mobile-responsive ePub books are available to download via the app. Most of our PDFs are also available to download and we're working on making the final remaining ones downloadable now. Learn more here.
Both plans give you full access to the library and all of Perlego’s features. The only differences are the price and subscription period: With the annual plan you’ll save around 30% compared to 12 months on the monthly plan.
We are an online textbook subscription service, where you can get access to an entire online library for less than the price of a single book per month. With over 1 million books across 1000+ topics, we’ve got you covered! Learn more here.
Look out for the read-aloud symbol on your next book to see if you can listen to it. The read-aloud tool reads text aloud for you, highlighting the text as it is being read. You can pause it, speed it up and slow it down. Learn more here.
Yes, you can access Creative Systems in Structural and Construction Engineering by Amarjit Singh in PDF and/or ePUB format, as well as other popular books in Tecnología e ingeniería & Ingeniería civil. We have over one million books available in our catalogue for you to explore.
Information
1 Keynote papers
Better constructed facilities through improved education
R.N. White
School of Civil and Environmental Engineering, Cornell University, Ithaca, N.Y., USA
ABSTRACT: This paper addresses a host of educational activities needed in the 21st century to ensure that we provide the best possible constructed facilities for the public and private sectors. Educational needs discussed here include specific improvements in how we teach structures to civil engineering undergraduates and graduate students; new emphases on materials engineering and selection; sustainability and life cycle issues; professional master’s programs; cultivating the “constructed facilities mentality”; continuing education at all levels and how the internet will make a huge difference; achieving a new level of mutual respect within the membership of the project team; and educating the owner. Each of these activities is aimed at providing better-constructed facilities with proper attention to actual life cycle costs, a healthy respect for the environment, and a renewed sensitivity about the aesthetic impact of everything we design and build.
1 Introduction and Scope of Coverage
This paper explores how education can be better utilized to achieve substantial improvements in designing and building all types of structures. “Improvements” should be assessed from the multiple perspectives of safety, serviceability, durability, constructability, esthetics, and lifetime cost. Discussion is aimed at new facilities as well as the rehabilitation of existing facilities.
This paper is based on the premise that improved and expanded education is more critical than ever before as we cope with construction needs in our crowded urban areas, utilizing a mix of new construction and rehabilitated existing infrastructure, with both types of construction utilizing improved “conventional” materials and a host of new materials. Owners face increasingly complex alternative scenarios with optimal solutions dependent upon a complex set of technical, technological, financial, social, environmental, and aesthetic issues. Implications for the detailed technical side of education are rather obvious, but we also must give expanded attention to our responsibilities in educating owners and clients.
As professionals, we collectively have the obligation to design and build structures and facilities to achieve an optimal blend of about a dozen parameters, including overall quality, safety, acceptable initial cost, economy of materials usage, durability, low maintenance, definable life cycle cost, appearance and aesthetics, impact on the external environment, internal environment of the facility occupants, and meeting all other expectations and needs of the owners. Some of these parameters complement each other and some are in direct contradiction. Hence the challenges to the project team — engineer, architect, materials supplier, contractor, sub-contractor, quality control and quality assurance personnel, maintenance organization, and owner — are daunting.
Meeting these challenges during the coming decades will provide almost unlimited opportunities for our young people, and we bear the responsibility of informing them about the excitement and challenges in the planning, engineering, and construction of tomorrow’s infrastructure. Unfortunately, our ability to attract the best and brightest young people into the civil engineering business seems to have suffered from the perception that we are an old-fashioned, overly mature industry. Let’s make some changes!
2 Basic Instruction in Design
2.1 Definition of issues
This discussion in this section is directed primarily at four-year B.S. degree programs in civil engineering, but this does not at all dilute the importance of our many excellent technology programs leading to Associate degrees or to the Bachelor of Technology. And given my background and experience, there is a natural bias towards coverage from the American perspective. But much of what I have to say applies internationally.
The scope, depth, and quality of structural design education varies considerably across our undergraduate and graduate programs in civil engineering. Civil engineers tend to be quite conservative, and as a profession we are slow to abandon old approaches and emphases developed over generations of teaching civil engineering students. Much of the constructed world around us shows that we have done quite well in the past, but many rather poor facilities also exist. The challenge is to devise strategies to do better! I believe there is urgent need for improvements in a number of key areas, including (a) preliminary design, (b) simple methods for estimating loads in members, (c) approximate design, (d) variability in loads and the influence of such factors as shrinkage and creep, (e) design of the entire structure, (f) the proper role of analysis in design, (g) effects of modeling assumptions on analysis results, (h) identification and control of errors encountered in design and construction, and (i) integration of materials selection and behavior into structural design and analysis processes.
2.2 Analysis and design of members and systems
We simply must teach more preliminary design, including simple methods for quickly estimating loads in members (slabs, beams, columns, etc.) and for sizing these primary components. Even modest coverage of approximate preliminary design can have a profound effect on a student, helping to develop an intuitive common sense about structures and giving a new sense of confidence about dealing with real structural design.
I recently taught undergraduate reinforced concrete structures in a reinforced concrete classroom building (one-way slabs supported on beam-and-column framing), and the very first assignment (given in the first lecture) was to have the students determine the amount of reinforcement needed at the center of the multi-span reinforced concrete beam spanning over the classroom. I provided the students with an absolute minimum of information: design live load, load factors for dead and live load, floor slab thickness, and reinforcement yield strength. They measured the actual structure for other dimensions. Many students struggled over this assignment, having to determine the load transfer paths, make meaningful estimates on bending moment values, and then reduce the flexural action to an internal couple approach with estimated location of the compressive resultant in the T-beam at midspan. But completion of the exercise was at a very high level of learning. It made later lecture coverage much more meaningful, particularly that on flexural strength of a section and calculation of bending moments and shears in frames.
The ability to quickly calculate approximate forces in any structure is also an absolutely necessary skill in checking the results of computer-based analysis and design. I advocate allocating at least 1/4 of the total time spent in teaching analysis to determining approximate forces. The sketching of approximate deflected shapes is an integral part of the approximate analysis.
Students deserve to get better explanations of the approximate nature of design, including the great variation in actual live loads, the real-life variability of material properties, and how poorly-defined effects such as shrinkage, support motions, unexpected restraining forces, and the like can often “overwhelm” the calculated effects of assumed loadings.
An obvious starting point is to use no more than two significant digits in expressing any final design calculation result. I cannot begin to count the times I’ve seen (in books, journals, and other publications) such quantities as M = 2, 248.9 m-kN or beam deflection = 4.187 mm. Students should be seeing these results as M = 2.3 m-mN and deflection = 4 mm. Without this first step, how can we possibly expect students to appreciate the fact that real-world design is not a precise science?
We must provide more emphasis on design of the entire structure, including the critical process of selecting the basic structural type to be used, and how the structural elements are connected. Perhaps the best way to accomplish this is to have a substantial portion of the total problem set assignments in the form of open-ended problems — rather simple structural situations at the undergraduate level and much more comprehensive and realistic projects at the graduate level (see Section 5). An example from the author’s own undergrad class at Cornell follows:
Working in teams of 3 engineers, we will be designing selected portions of a three-stoiy general-purpose academic building with a footprint of 5000 square feet and a total gross floor area of 15,000 square feet, to be located in the current parking lot behind Thurston Hall. The building is to have a full basement, a freight elevator, and two sets of stairs. In this first assignment, you are to propose a typical floor framing plan (location of columns, definition of slab spans and supporting beams and girders). Clear height between top of floor and lowest part of underside of floor framing above is to be 10 feet. Live load is to be 125 psf (conservatively selected to permit changing use of the building space by different groups). Material strengths are to be selected by each team.” In subsequent assignments the building design was refined, with final design being completed for all typical elements of the building, including foundations.
The proper role of analysis in the design process needs better definition. We need to continually stress that the prime role of analysis is to improve design and hence improve the overall quality of the completed project. Student attitudes on any subject correlate well with faculty attitudes. There is far too much separation of analysis and design in academia; often we see one group of faculty teaching analysis and another group teaching design. A solution is pretty obvious! However, if tradition or some other reason still dictates that separate courses be given, then it is essential to have some modest coverage of the behavior of steel and concrete structures prior to teaching analysis, in order to have realistic and meaningful discussions on effective stiffness of members and behavior at loads beyond the proportional limits.
Effects of assumptions on types of supports, boundary conditions, foundation stiffness, and the like need to be integrated into the lecture material and in the problem assignments. Students too often begin to think that supports are either hinged or fixed, and that building frames stop at ground level -- a rather ridiculous state of affairs considering real-world conditions. Fundamental concepts on foundations should be integrated into the structures classes, with continual linkages between courses in structures and in soil mechanics and foundations.
At the upper-class level, and in graduate education in structural engineering, we need to convey the sensitivity of results (forces and displacements) to the modeling assumptions involved in the analysis, particularly the more complex problems that utilize finite element approaches. Behavior beyond the elastic limit is particularly sensitive to material properties, mesh fineness, and load increment size. An effective approach is to have students (or teams of students) model and analyze the same structure, using the finite element approach, and then compare results and critically discuss the effects of different modeling assumptions.
Students should have at least a minimal understanding of errors in design and construction — the most typical errors and mistakes, potential impact on safety and serviceability, checking processes, and error control in the office and in the field. A single lecture on this topic, supplemented by readings from Nowak 1986, will suffice as long as the topic is pursued further at appropriate times in other courses. My experience indicates that students become highly motivated in these discussions ~ the topic is perfect for problem assignments given to teams of three or four students, followed by class discussions.
2.3 Integration of materials into structural analysis and design
The specification of proper materials and the integration of the material properties into all phases of the analysis and design process may well be the most critical step in arriving at a durable, top-quality constructed facility. Some brief comments on how materials might be best integrated into the teaching of structural design will be given here, with the major coverage on materials to follow in Section 3 below.
In contrast to modern design practice, where the individual project needs dictate the choice of materials, academia has tended to have separate courses on the design of concrete, steel, timber, and masonry structures. There are certain advantages of immersing students in, say, steel structures design exclusively for several months, and then follow up with a similar immersion in concrete structures design. But at some point some merging of materials needs to be done. I suggest that the first course in structural engineering have a limited coverage of the primary issues met in designing and building with a variety of materials, with later courses having more concentrated coverage, and perhaps the final course going back to “general design” where the students have to make the decisions on which material(s) to use. And certainly graduate level design should have substantial components of “multi-material” design, a topic to be discussed more in Section 5 below.
As an example of how most designs incorporate two or more primary construction materials, most modern high-rise buildings now utilize some type of composite (hybrid) framing to carry gravity loads and lateral forces (wind and seismic). Composite construction utilizes concrete and steel, each to its best advantage, providing a fire-resistant, economical structural system that is fast to construct and provides substantial stiffness to resist lateral drift. The extensive activity in composite construction (Xiao & Mahin 2000) is expected to continue, with an ever-increasing usage of high strength concrete and high-performance structural steel, and with extensions to buildings of medium and moderate height. One of the greatest challenge in designing and building a high-rise composite framed building is developing a strategy that will guarantee reasonably flat floors after time-dependent deformations in the columns have essentially stabilized. This requires a level of knowledge about the concrete in the columns that far exceeds what we typically know about concrete in the usual structure.
2.4 How do we fit everything into the curriculum?
This is an age-old problem because there is always more to teach than time permits, particularly when an ever-expanding list of important topics needs coverage (e.g., computer science, materials science, probability and statistics, systems engineering, environmental issues, costs and economics, etc.). The apparent solution is to decrease existing coverage at the B.S. level and then rely on students to continue their study at the Master’s level, either full-time or part-time while working, or in on-the-job training. The undergraduate courses then must strive to teach the fundamentals in an integrated fashion, and to teach the students how to think critically and how to learn.
Economies in coverage can begin early, say during the teaching of mechanics of materials, where problems should be designed to illustrate practical situations, with later courses building directly on the mechanics coverage. We probably spend more time on flexure than is really needed; it is a “comfortable” topic with results conforming very well with basic assumptions. Teaching flexure from the standpoint of two internal forces (C and T) separated by an internal moment arm provides a fundamental basis that can be then readily adapted to any material and to both elastic and inelastic behavior. It also helps in teaching shear, with horizontal shear stress resulting from differe...
Table of contents
- Cover
- Half Title
- Title Page
- Copyright Page
- Table of Contents
- Preface
- Acknowledgements
- Scientific and Technical Committee
- 1 Keynote papers
- 2 Ethics and education
- 3 Housing and architecture
- 4 Constructability and design-construction
- 5 Construction methods
- 6 Construction contracting and practice
- 7 Performance improvement
- 8 Procurement and project management
- 9 Cost Systems – I
- 10 Cost Systems – II
- 11 Planning and knowledge management
- 12 Information technology
- 13 Safety and quality
- 14 Concrete mixes
- 15 Material enhancement
- 16 Concrete material behavior
- 17 Soils and pavement analysis
- 18 Structural design issues
- 19 Computer algorithms and fuzzy theory applications
- 20 Structural analysis by special methods
- 21 Structural behavior of structural elements
- 22 Structural dynamics
- 23 Seismic response of structural elements
- 24 Mechanical behavior
- 25 Concrete shear
- 26 Steel structures
- 27 Structural behavior of bridges
- 28 Structural behavior of plates and slabs
- 29 Inelastic behavior of structures
- 30 Mechanical behavior of damaged structures
- Author index