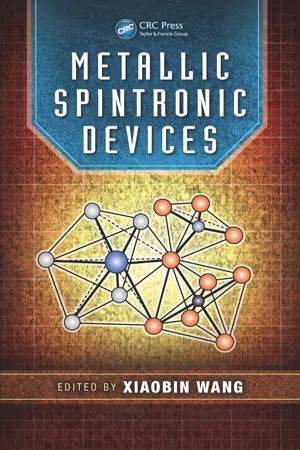
This is a test
- 274 pages
- English
- ePUB (mobile friendly)
- Available on iOS & Android
eBook - ePub
Metallic Spintronic Devices
Book details
Book preview
Table of contents
Citations
About This Book
Metallic Spintronic Devices provides a balanced view of the present state of the art of metallic spintronic devices, addressing both mainstream and emerging applications from magnetic tunneling junction sensors and spin torque oscillators to spin torque memory and logic. Featuring contributions from well-known and respected industrial and academic experts, this cutting-edge work not only presents the latest research and developments but also:
- Describes spintronic applications in current and future magnetic recording devices
- Discusses spin-transfer torque magnetoresistive random-access memory (STT-MRAM) device architectures and modeling
- Explores prospects of STT-MRAM scaling, such as detailed multilevel cell structure analysis
- Investigates spintronic device write and read optimization in light of spintronic memristive effects
- Considers spintronic research directions based on yttrium iron garnet thin films, including spin pumping, magnetic proximity, spin hall, and spin Seebeck effects
- Proposes unique solutions for low-power spintronic device applications where memory is closely integrated with logic
Metallic Spintronic Devices aims to equip anyone who is serious about metallic spintronic devices with up-to-date design, modeling, and processing knowledge. It can be used either by an expert in the field or a graduate student in course curriculum.
Frequently asked questions
At the moment all of our mobile-responsive ePub books are available to download via the app. Most of our PDFs are also available to download and we're working on making the final remaining ones downloadable now. Learn more here.
Both plans give you full access to the library and all of Perlego’s features. The only differences are the price and subscription period: With the annual plan you’ll save around 30% compared to 12 months on the monthly plan.
We are an online textbook subscription service, where you can get access to an entire online library for less than the price of a single book per month. With over 1 million books across 1000+ topics, we’ve got you covered! Learn more here.
Look out for the read-aloud symbol on your next book to see if you can listen to it. The read-aloud tool reads text aloud for you, highlighting the text as it is being read. You can pause it, speed it up and slow it down. Learn more here.
Yes, you can access Metallic Spintronic Devices by Xiaobin Wang in PDF and/or ePUB format, as well as other popular books in Physical Sciences & Physics. We have over one million books available in our catalogue for you to explore.
1
Perpendicular Spin Torque Oscillator and Microwave-Assisted Magnetic Recording
Jian-Gang (Jimmy) Zhu
Data Storage Systems Center, Department of Electrical and Computer Engineering, Carnegie Mellon University, Pittsburgh, Pennsylvania
CONTENTS
1.1 Introduction
1.2 Perpendicular Spin Torque Oscillator Design Free of External Field
1.3 Microwave-Assisted Magnetic Recording
Acknowledgment
References
1.1 Introduction
For more than 100 years, virtually all electronic devices had been operated by moving, accumulating, and storing charges carried by either electrons or holes. Spin, the other important property possessed by electrons and holes, besides charge, had largely been ignored in practical applications—until the late 1980s, when the first magnetoresistive sensor based on anisotropic magnetoresistance (AMR) effect was used as a read head in a hard disk drive (HDD) [1]. The magnetic fields from recorded bits in a magnetic thin-film medium was sensed in a thin magnetic film element through a direct resistance change other than measuring magnetic induction that has been used ever since Faraday’s 1831 experiment. Accompanied by the excitement from the perspective of commercial technology application, the discovery of the giant magnetoresistance (GMR) effect in 1988 reignited the field of magnetism [2]. The rapid commercialization of the GMR-based read sensor in hard disk drives in the mid-1990s firmly convinced the world that the field of spinbased electronics had begun [3, 4].
One of the fundamental physics concepts behind magnetoresistance effects is the fact that an electron current carries a net spin angular momentum after passing through a magnetized ferromagnetic film. When a spin-polarized current interacts with a local magnetization by exerting a torque on the localized spins, referred to as spin-transfer torque (STT), the orientation of the magnetization could be altered. Following pioneering theoretical work [5, 6], an experimental demonstration in 1999 showed the world for the first time that a spin-polarized current, other than a magnetic field, can be used to switch the magnetization of a ferromagnetic film [7], as many other experiments showed later [8, 9]. Magnetoresistive random access memory (MRAM) based on the STT effect, referred to as STT-MRAM, was quickly proposed along with research and development efforts [10,11,12,13,14,15,16, and 17]. An MgO-based magnetic tunnel junction has been used as a memory element for both the in-plane magnetization mode and perpendicular magnetization mode [18,19, and 20].
It has also been proposed [21] and experimentally investigated that STT can excite spin waves by facilitating spin precession [8, 22,23, and 27]. The effect was then quickly utilized to build current-driven magnetic oscillators, with in-plane magnetization mode [24] and perpendicular magnetization mode [25, 26, 28, 29, 30, and 31], as well as various alternative modes [32, 33].
In this chapter, a design of the perpendicular spin torque oscillator will be reviewed [28]. In particular, its application as a core component for a novel technology, referred to as microwave-assisted magnetic recording, potentially for future hard disk drives, will be discussed in detail [34].
1.2 Perpendicular Spin Torque Oscillator Design Free of External Field
In the perpendicular spin torque oscillator design shown in Figure 1.1, the multilayer stack consists of a spin polarization layer of sufficient perpendicular anisotropy, a nonmagnetic interlayer that is either a metallic or tunnel barrier, and the oscillation layer magnetically coupled to another magnetic layer with perpendicular anisotropy.
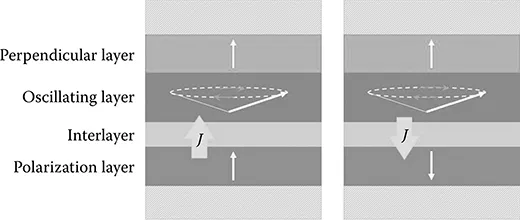
FIGURE 1.1 Schematic drawing of the perpendicular spin torque oscillator design in two oscillating modes, in which the chirality of the magnetization precession in the oscillating layer is solely determined by the magnetization of the perpendicular layer.
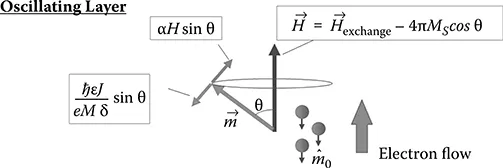
FIGURE 1.2 Spin-transfer torque from spin-polarized current generates an antidamping torque and creates a nonequilibrium condition for the magnetization orientation, yielding a steady gyromagnetization precession of the magnetization in the oscillating layer. (From X. Zhu and J.-G. Zhu, IEEE Trans. Magn., 42(10), 2670–2672, 2006. With permission.)
Electron current flow through the polarization layer carries net spin angular momentum. The transfer of the angular momentum in the oscillating layer generates a torque on the local magnetization, tilting the magnetization away from the perpendicular direction provided the current density is sufficient. As illustrated in Figure 1.2, at the new balance between the damping torque and the spin-transfer torque, the magnetization no longer is aligned with the local effective magnetic field. The magnetization then naturally precesses around the effective field while maintaining a constant tilting angle at constant current density. The STT-included Landau-Lifshitz-Gilbert equation for describing the gyromagnetic motion of the magnetization in the oscillating layer [6] can be written as
where the effective magnetic field, H, consists of the exchange coupling field arising at the interface with the perpendicular layer and the self-demagnetization field, which depends on the tilting angle θ:
The precessional frequency is then solely determined by the effective field:
Assuming the exchange coupling field is sufficiently greater than the selfdemagnetization field, the oscillation will not start until the current density is sufficient to yield a nonzero magnetization tilting angle. The critical current density to yield a steady precession is
For current density above the critical current density Jc , we have the following linear relationship with current density:
The above equation shows that the oscillating frequency can be tuned by the current amplitude and linearly increases with increasing current density. This frequency-tunable feature with varying current alone makes this spin torque oscillator extremely interesting for potential technology applications, and the perpendicular spin torque oscillator unique in contrast to that of the in-plane type of spin torque oscillators.
The frequency-tunable range by varying the current is
Keep in mind that the above relation is obtained with the assumption that the magnetization of the perpendicular layer is assumed to be always in the perpendicular direction due to sufficiently strong perpendicular anisotropy in the absence of current. In reality, the frequency-tunable range will be reduced if the perpendicular layer tilts away from the perpendicular direction during the current application.
Micromagnetic modeling studies, which include finite size effect as well as the magnetostatic interactions between all the magnetic layers, have shown some very interesting properties. Figure 1.3 shows simulated STT-driven magnetization precession for a perpendicular spin oscillator design with the perpendicular layer of very high magnetocrystalline anisotrop...
Table of contents
- Cover Page
- Title Page
- Copyright Page
- Contents
- Preface
- About the Editor
- Contributors
- 1 Perpendicular Spin Torque Oscillator and Microwave-Assisted Magnetic Recording
- 2 Spin-Transfer-Torque MRAM: Device Architecture and Modeling
- 3 The Prospect of STT-RAM Scaling
- 4 Spintronic Device Memristive Effects and Magnetization Switching Optimizing
- 5 Magnetic Insulator-Based Spintronics: Spin Pumping, Magnetic Proximity, Spin Hall, and Spin Seebeck Effects on Yttrium Iron Garnet Thin Films
- 6 Electric Field-Induced Switching for Magnetic Memory Devices
- Index