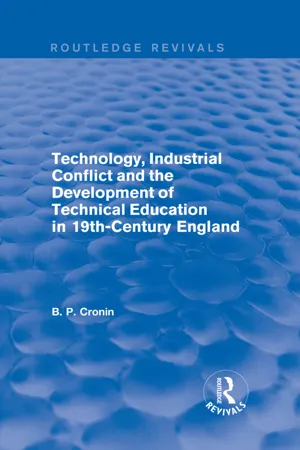
eBook - ePub
Technology, Industrial Conflict and the Development of Technical Education in 19th-Century England
This is a test
- 316 pages
- English
- ePUB (mobile friendly)
- Available on iOS & Android
eBook - ePub
Technology, Industrial Conflict and the Development of Technical Education in 19th-Century England
Book details
Book preview
Table of contents
Citations
About This Book
This title was first published in 2001. Nineteenth-century employers played a crucial role in the training and education of young workers in England. This multi-disciplinary study traces the connection between problems of technical education development and the increasingly antagonistic relations with skilled workers, culminating in the Great Strike and Lockout of 1897. Cronin demonstrates that employers, dominated by economic short-termism, extended their hegemony beyond the boundaries of the factory gates. Their reluctance to endorse and sponsor technical education radically influenced the perception of technical education held by government and local authorities.
Frequently asked questions
At the moment all of our mobile-responsive ePub books are available to download via the app. Most of our PDFs are also available to download and we're working on making the final remaining ones downloadable now. Learn more here.
Both plans give you full access to the library and all of Perlego’s features. The only differences are the price and subscription period: With the annual plan you’ll save around 30% compared to 12 months on the monthly plan.
We are an online textbook subscription service, where you can get access to an entire online library for less than the price of a single book per month. With over 1 million books across 1000+ topics, we’ve got you covered! Learn more here.
Look out for the read-aloud symbol on your next book to see if you can listen to it. The read-aloud tool reads text aloud for you, highlighting the text as it is being read. You can pause it, speed it up and slow it down. Learn more here.
Yes, you can access Technology, Industrial Conflict and the Development of Technical Education in 19th-Century England by Bernard P. Cronin in PDF and/or ePUB format, as well as other popular books in History & World History. We have over one million books available in our catalogue for you to explore.
Chapter One
Nineteenth-century machine tools: the work of Maudslay, Nasmyth and Whitworth
The basis of the transformation of engineering processes
It is well documented that important changes in the technical mode of production were initiated in the 1850s, and earlier, by engineer-entrepreneurs such as Muir, Maudslay, Fairbairn, Nasmyth and Whitworth (Smiles 1876, Roe 1916, Clapham 1938, Chadwick 1958, Galloway 1958, Steeds 1970, Cardwell 1972, McGufFie 1986). Early technological innovations were also closely related to changing strategies of labour control, clearly illustrated by Whitworth's work on precision measurement and standards1 in engineering and manufacturing production.
The deployment of innovatory machine tools and technological factors, exemplified by Whitworth's research into metrology, are conventionally perceived as evolutionary developments in new classes of machines and of new metrological precision. His technological innovations also entailed the reorganisation of technical and labour control in the workshops and may be realistically considered as significant factors in changing employer-employee relations and the reorganisation of work.
The standards of measurement and gauging, with which Whitworth was deeply engaged, were fundamental to the creation of new levels of workshop precision. An often neglected aspect of his pioneering work in this factor of production was his contribution to the decline of the apprenticeship system by encouraging the principle of substitution. This is not a denigration of his exemplary foresight in sponsoring the education of young engineers through the 'Whitworth Scholarships'. The decline of the apprenticeship system reflected the employers' increasing control over skilled labour and precision manufacture particularly, and a growing distrust of the craft apprenticeship form of training. There are important parallels to be drawn between the substitution of parts (interchangeability based on fixed standards of measurement) and the substitution of labour (part of the general process of 'de-skilling' of jobs and workers).
The specifics of engineering production, such as new techniques of precision measurement and control of rapid metal removal in cutting operations, were highly significant for employers in their attempts to control skilled labour. Any account of 19th-century technical change cannot be separated from analysis of the major employers' concern to transform the organisation of engineering work and extend their control over the planning and execution of the total labour process.
The machining of metals was a difficult and expensive process if accuracy was specified. Much depended upon the skill of the artisan and the basic design and structure of the machine tools. It was common up to the middle of the 19th century to view the self-activity of the skilled workers as autonomous, particularly in relation to the planning and execution of production processes. The artisan's traditional function of production control was increasingly curtailed by the employers' implementation and control of new technologies after the 1850s. There was thus enduring artisan resistance to management reorganisation of work.
Historically, skilled workers had demonstrated their 'indispensability' by working with speed and accuracy. In practice this meant that employers were forced to rely upon artisans to produce work to 'acceptable' tolerances in a manufacturing situation in which there were no universal standards governing levels of accuracy. The issue for management was to find strategies, and the technology, with which to replace essential artisan skills. Whitworth's insight was to recognise that employer dominance would always be undermined without control over machine-tool accuracy and production. His research into 'standards' clearly influenced subsequent technological developments, both in machine tools and metrology. Technical and social control of the workplace underwent fundamental changes, effecting changes beyond the workshop such as attitudes towards technical training, craft apprenticeships, and the employment of child labour generally, and the foundation of a formal and separate technical education system based predominantly on a part-time principle.
Apart from generalised workshop skills there were specific artisan attributes that extended beyond the banausic, the 'art' of engineering bridging the gap between science and the engineering product: 'The creative, constructive knowledge of the engineer is the knowledge needed to implement that "art" (Vincenti 1990: 4). Two such skill elements were evident in the 19th-century workshop: a working knowledge of common metals used throughout the period; and a thorough understanding of the capabilities of a whole range of machine tools.
Common nineteenth-century materials used in engineering production
Maximum metal-cutting speeds depend mainly upon the material being cut and the metallurgical composition of the cutting tool. The principal material for cutting metals throughout the 19th century was plain carbon steel. The essential difference between cast iron, a common fabricating material of the period, and steel was the amount of carbon contained in the constituency of the metal. Pure iron, called ferrite, is a soft metal having a structure composed of crystals or grains. Cast iron contained approximately 92-4 per cent iron and about 3.5 per cent carbon, plus some small quantities of constituents such as silicon (2%), manganese (1%), sulphur (0.1%) and phosphorous (0.3-1.2%) (Chapman 1943: 12).
Steel is fundamentally an alloy of iron and carbon with the content of carbon varying from 0.1 up to a maximum of 2.5 per cent. If the carbon content is increased beyond this level, excess carbon is distributed throughout the material in the form of free graphite. Further increases would merge the metal into the group of metals termed the cast irons. Thus the crucial constituent in plain carbon steels was the presence of carbon up to about 1-2 per cent only. For a material to be classed as a steel there must be no free graphite (as in cast iron) in its composition. (Sportn's Dictionary 1873: 2921). As a general guide the plain steels (as distinct from complex alloys, which were developed late in the nineteenth century) were classified according to their carbon content (see Table 1.1).
Table 1.1 Common 19th-century steels
Steel | % Carbon | Uses |
dead mild | 0.1-0.125 | wire rod, sheets, tubes |
mild | 0.15-0.3 | boiler plates, bridge work, drop forgings, general workshop use |
medium | 0.3-0.5 | axles, drop forgings, agricultural tools |
carbon | 0.5-0.7 | springs, locomotive tyres, hammers |
high | 0.7-0.9 | springs, shear blades, chisels |
carbon | 0.9-1.1 | press dies, punches, screwing dies, axes, picks |
1.1-1.4 | razors, files, drills, gauges, metal-cutting tools |
Source: Chapman (1943), p. 16.
Preparation and cutting sequence of metal-cutting tools
The geometry of metal-cutting tools and the cutting sequence of machine-tool operation would normally follow this pattern:
In order to remove surplus metal from the material being cut, the 'stock', the cutting tool was first forged by the machinist, that is, shaped from the raw metal in the blacksmith's fire. Then the basic tool shape was ground (shaped to size using a standard grinding or emery wheel) to produce the necessary

Fig. 1.1 A typical straight-edge lathe tool (left hand)
cutting angles (Spon's dictionary (1873), p. 2921). These angles vary according to the material being cut and the tool composition; in the period up to the late 1880s this tool material was known as plain carbon steel. The composition and metallurgical properties of this metal had a critical bearing on the rate at which various metals could be cut - the standard tool-preparation rule of the period was that the harder the material being machined, the greater the wedge angle and the smaller the clearance angle. The machinist was responsible for forming and maintaining the correct angles on the cutting tool; the main objective was to keep the tool edges sharp enough to slice through the metal, with a wedge angle sufficient to provide strength, and a minimum of clearance to prevent overheating from friction due to the cutting forces. Figure 1.1 shows the standard tool of the period.
It may be noted that, in general, the toughness, tenacity, and hardness of steel increase with the quantity of carbon it contains.
Apart from the cutting-tool angles, two other critical metal-cutting variables were determined by the machinist: the depth of cut (the amount of penetration into the workpiece by the cutting tool), and the feed rate (the amount the tool travels per revolution or 'pass'). Once these variables had been determined, the depth of cut (which depends upon material being cut and the cutting-tool material) was applied, often by use of a micrometer wheel, and an appropriate feed rate through the self-acting mechanism activated. This latter function employed the use of a lever to engage a nut housed in the saddle, carrying the slide rest, supporting the cutting tool, with the leadscrew driven by a gear train ('cogs' in mesh, i.e. driving and being driven).
Box 1.1 shows the arithmetic involved in calculating the gears required for thread-cutting, 'meshing', in order accurately to determine the correct ratio of turns of the work to linear movement of the tool. Referring to a lathe, the gear train was arranged so that the headstock, with a gripping mechanism, a 'chuck', carrying the material being cut, revolved in a constant ratio movement relative to the travel of the cutting tool. This means that, in the case of a centre lathe, the work revolves at a predetermined rate (revolutions per minute, r.p.m.) whilst the cutting tool advances a constant amount parallel or at right angles to the axis of the work (in inches per revolution).
Lathes were available with longitudinal and cross-feed motions driven by gears and the rack (a long 'screw', flat on one side and fixed to the bed of the machine), giving 16 changes of feed to each. The operator had only to secure a sliding arm to the proper figure on an index stud and the machine would cut the pitch indicated by the figure (Bement, Mills & Co. 1893: 29). As ea...
Table of contents
- Cover
- Half Title
- Title
- Copyright
- Contents
- List of figures, boxes and tables
- Preface
- Abbreviations
- Introduction
- 1 Nineteenth-century machine tools: the work of Maudslay, Nasmyth and Whitworth
- 2 The interaction of work and technology: the influence of F. W. Taylor and H. Braverman
- 3 Technology, changing definitions of skill and the use of child labour
- 4 Skill and the collapse of the craft apprentice system
- 5 Employers and the ideology of engineering management: the Great Strike of 1897-8 and its aftermath
- 6 Contradictions and struggle in ideas about a national system of technical education
- 7 Employers, the foundation of the City and Guilds of London Institute and government legislation
- 8 Industrial conflict and 19th-century technical and educational change
- References and Bibliography
- Index