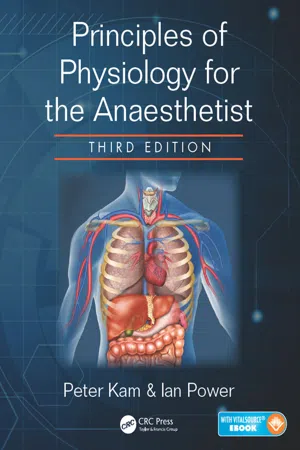
This is a test
- 500 pages
- English
- ePUB (mobile friendly)
- Available on iOS & Android
eBook - ePub
Book details
Book preview
Table of contents
Citations
About This Book
Principles of Physiology for the Anaesthetist, now in its Third Edition, continues to provide candidates with a "tailor-made" alternative to more general physiology textbooks and delivers information designed and written specifically with the trainee anaesthetist in mind. The book covers the physiology of all major organ systems, with specific emph
Frequently asked questions
At the moment all of our mobile-responsive ePub books are available to download via the app. Most of our PDFs are also available to download and we're working on making the final remaining ones downloadable now. Learn more here.
Both plans give you full access to the library and all of Perlegoâs features. The only differences are the price and subscription period: With the annual plan youâll save around 30% compared to 12 months on the monthly plan.
We are an online textbook subscription service, where you can get access to an entire online library for less than the price of a single book per month. With over 1 million books across 1000+ topics, weâve got you covered! Learn more here.
Look out for the read-aloud symbol on your next book to see if you can listen to it. The read-aloud tool reads text aloud for you, highlighting the text as it is being read. You can pause it, speed it up and slow it down. Learn more here.
Yes, you can access Principles of Physiology for the Anaesthetist by Peter Kam, Ian Power in PDF and/or ePUB format, as well as other popular books in Medicine & Medical Theory, Practice & Reference. We have over one million books available in our catalogue for you to explore.
Information
1
Physiology of excitable cells
LEARNING OBJECTIVES
After studying this chapter, the reader should be able to
1. Describe the nature of the cell membrane and the ionic basis of the resting membrane potential.
2. Describe the nerve action potentials in nerves, cardiac cells and muscle cells.
3. Describe the propagated action potential.
4. Understand ion channels that are voltage gated: sodium, potassium and calcium channels.
5. Describe neurotransmitters and receptors, including ion channels, G proteins and second messengers.
6. Understand the structure and function of the specialized connection between nerves and striated muscle (the neuromuscular junction), with comprehension of the mechanism of neuromuscular transmission.
7. Understand the different structures of skeletal, cardiac and smooth muscles.
8. Describe the role of muscle spindles, Golgi tendon organs and spinal reflexes.
9. Understand the classification and mechanisms of activation of sensory receptors, including those for pain.
INTRODUCTION
An excitable cell responds to a stimulus by a rapid change in the electrical charge of the cell membrane. Nerve, muscle and sensory cells can be included in this classification, as the excitability of these tissues lies in the changing electrical properties of their membranes.
This chapter examines the physiology of some excitable cells. First, the structure of the cell membrane and the ionic basis for the resting membrane potential are examined, followed by the form of action potential for both nerves and muscle. Next, voltage-sensitive membrane ion channels are described and their role in the action potential is discussed. In the section âMembrane potentialâ, chemical communication between excitable cells is examined, by investigating the interaction of receptors, ion channels, G proteins and intracellular messengers. The physiology of neuro-muscular transmission is then presented, followed by a discussion of skeletal, cardiac and smooth muscle cells, together with details of contractile proteins, the mechanical response to stimulation and excitationâcontraction coupling. In the section âMembrane potentialâ, organs involved in the regulation of muscle movement, muscle spindles and tendon organs are discussed, together with an examination of stretch reflexes. Finally, sensory receptors are classified and their physiology is described.
MEMBRANE POTENTIAL
Cell membrane
Cell membranes (Figure 1.1) are bilayers of phospholipids with polar heads to the outside and Physiology of excitable cells lipophilic fatty tails to the inside. This structure separates the cellular contents and the cytoplasm from the extracellular fluid. Integral proteins traverse the membrane, whereas peripheral proteins sit on the cytoplasmic and extracellular surfaces. Peripheral proteins on the inside and outside have different functions; glycoproteins have their sugar residues on the outside, and transporting enzymes bind adenosine triphosphate (ATP) on the inside.
The cell membrane can be thought of as a layer of insulation covered on both sides by conducting material. This structure is traversed by protein channels that determine ionic permeability and the resultant electrical potential across the membrane.
RESTING MEMBRANE POTENTIAL
Cell membranes have an electrical charge across them with the inside being 70â80 mV negative with respect to the outside (Figure 1.2). This electrical potential depends on two main factors: the selective membrane permeability to ions and the different ionic concentrations on the inside and outside of the cell. The former is the more important factor, as it can change. At rest, most membranes are permeable to potassium but not sodium ions. Membranes can be described as being semipermeable to ions; potassium passes across them with ease, but most others do not (Figure 1.3).
The potassium concentration is much higher inside (150 mM) than outside (5 mM) the cell. Potassium diffuses down this concentration gradient out of the cell, but this movement cannot continue indefinitely as it is opposed by electrical forces. As the membrane is impermeable to other cations, the movement out by positively charged potassium ions produces a negative charge on the inside of the cell. This charge tends to oppose and prevent further movement of potassium out of the cell. Therefore, there are opposing chemical and electrical gradients acting on potassium ions (chemical: out; electrical: in). At the resting membrane potential of around â70 mV, the electrical and chemical gradients acting on potassium ions balance. That is, the resting membrane potential is due to the equilibrium of the electrochemical gradients affecting potassium ions (Figure 1.4).
The Na+ âK+ pump, which transports three sodium ions to the outside for every two potassium ions pumped inside, causes a continual loss of positive ions from inside the membrane and therefore is electrogenic because it produces a net deficit of positive charges inside the cell. This causes an additional negative charge of about â4 mV inside the cell membrane.
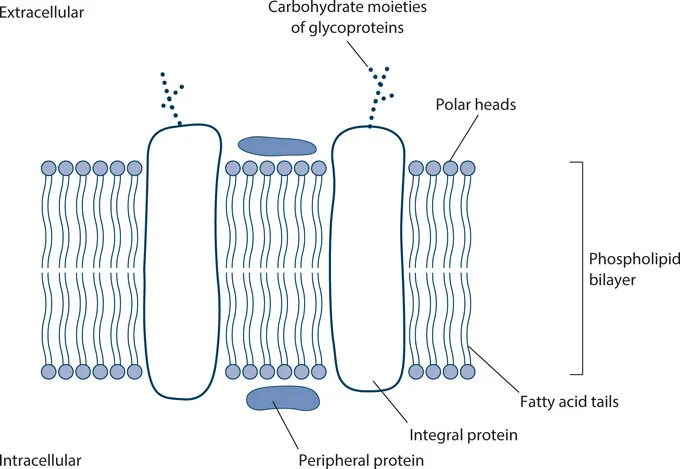
Figure 1.1 Diagrammatic representation of the cell membrane.

Figure 1.2 The resting membrane potential.

Figure 1.3 Membrane permeability to sodium and potassium ions. (The chemical symbol size indicates the relative ionic concentrations of K+ and Na+.)
It is important to realize that only a small number of potassium ions have to move to produce the resting potential. For a membrane potential of â70 mV, the difference between the numbers of positive and negative charges inside the cell is only 0.000002%.
Proteins are the main intracellular anions, but they also have little effect as they cannot traverse the membrane. The primary extracellular ion â chloride â can pass freely across the membrane (Figure 1.5), but the resultant potential is similar to that set up by potassium. This can be demonstrated by considering the electrochemical forces on chloride ions.

Figure 1.4 Equilibration of potassium ions across the cell membrane â the electrochemical gradient. At the equilibrium potential for potassium, the chemical and electrical gradients are in balance. Îc, chemical gradient; Îe, electrical gradient.
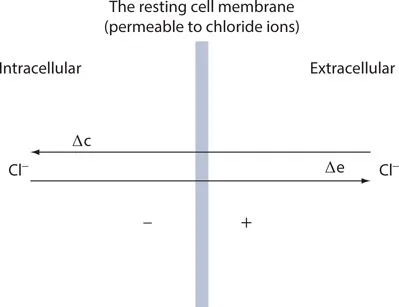
Figure 1.5 Passage of chloride ions across the cell membrane.
Other cations â sodium included â contribute relatively little to the resting potential as the membrane is quite impermeable to them. If the membrane was permeable to sodium, then the potential would be completely reversed as the electrochemical gradient is opposite to that of potassium (Figure 1.6).

Figure 1.6 Passage of sodium ions across the cell membrane.
Nernst equation
The Nernst equation calculates the potential difference that any ion would produce if the membrane was permeable to it...
Table of contents
- Cover
- Half Title
- Title Page
- Copyright Page
- Table of Contents
- Preface to the first edition
- Preface to the second edition
- Preface to the third edition
- Authors
- Contributors
- 1 Physiology of excitable cells
- 2 Physiology of the nervous system
- 3 Respiratory physiology
- 4 Cardiovascular physiology
- 5 Gastrointestinal physiology
- 6 Liver physiology
- 7 Renal physiology
- 8 Acidâbase physiology
- 9 Physiology of blood
- 10 Physiology of the immune system
- 11 Endocrine physiology
- 12 Metabolism, nutrition, exercise and temperature regulation
- 13 Physiology of pain
- 14 Maternal and neonatal physiology
- 15 Physiology of ageing
- 16 Special environments
- Key equations and tables
- Further reading
- Index