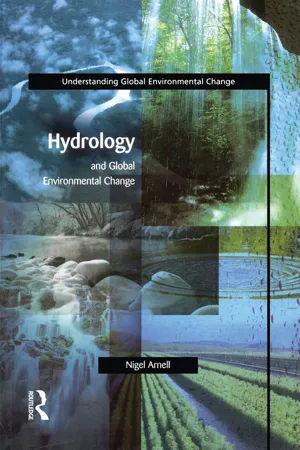
This is a test
- 364 pages
- English
- ePUB (mobile friendly)
- Available on iOS & Android
eBook - ePub
Hydrology and Global Environmental Change
Book details
Book preview
Table of contents
Citations
About This Book
Hydrology and Global Environmental Change presents the hydrological contribution to, and consequences of, global environmental change. Assuming little or no prior knowledge on the part of the reader, the book looks at the main processes of global environmental change - global scale processes, large regional processes, repetitive processes - and how the hydrological cycle, processes and regimes impact on GEC and vice-versa.
Frequently asked questions
At the moment all of our mobile-responsive ePub books are available to download via the app. Most of our PDFs are also available to download and we're working on making the final remaining ones downloadable now. Learn more here.
Both plans give you full access to the library and all of Perlego’s features. The only differences are the price and subscription period: With the annual plan you’ll save around 30% compared to 12 months on the monthly plan.
We are an online textbook subscription service, where you can get access to an entire online library for less than the price of a single book per month. With over 1 million books across 1000+ topics, we’ve got you covered! Learn more here.
Look out for the read-aloud symbol on your next book to see if you can listen to it. The read-aloud tool reads text aloud for you, highlighting the text as it is being read. You can pause it, speed it up and slow it down. Learn more here.
Yes, you can access Hydrology and Global Environmental Change by Nigel W. Arnell in PDF and/or ePUB format, as well as other popular books in Ciencias físicas & Geografía. We have over one million books available in our catalogue for you to explore.
Information
Chapter 1
Hydrology, earth system science and global environmental change
1.1 Introduction
The year 2000 saw significant flooding in many parts of the world. The largest floods for many decades struck Mozambique in February, driving 733 000 people from their homes and killing 929. Over four million people living in the Mekong Delta were affected by a series of floods between September and November - the largest for 70 years - and 865 people lost their lives. Nearly 1500 people in eastern India and Bangladesh were killed by floods in September and October, and up to 24 million people were displaced. In Europe, the largest floods for at least 50 years inundated many floodplains in northern Italy during October, and Britain experienced the most widespread flooding in October and November for more than 50 years: river levels at York rose to their highest level since 1625.
After each of these floods - and indeed after most of the largest floods during the last decade of the twentieth century - journalists, the public and politicians have speculated that various human activities may be making floods both more frequent and more severe. Was the unusually heavy rainfall that triggered many of the floods of 2000 “caused” by global warming? Were the floods exacerbated by land use change in the catchment, such as urbanisation and deforestation? Were flood heights increased because of the construction of flood banks upstream? To what extent was the increased flood damage due to increased exposure to flood loss? Was the year 2000 really any worse than previous years?
Floods are just one of the ways in which human society interacts with the hydrological system. People need water for drinking, washing and preparing food, farmers need water to irrigate crops, industry uses water both as a raw material and for cooling, and rivers are important parts of the transport network in many countries: rivers are also used to carry away waste. Pressures on the available water are increasing in many countries, as demand for water rises, and at the same time the resource is often being polluted and degraded. Polluted water can carry disease and lead to ill-health and death, and the extent and timing of many water-borne diseases - such as malaria and schistosomiasis are very much influenced by hydrological regimes.
Activities in the catchment, such as deforestation, urbanisation, abstraction of water and the use of agricultural chemicals, are also affecting hydrological processes and regimes - and these effects have an impact on the water environment, not only in the river channel but also in wetlands, on the floodplain and along the river corridor. Human activities are also affecting the inputs to the catchment, through global warming and acid rain. The United Nations Environment Program’s review of the state of the world’s environment (UNEP, 1999) highlights many examples of the degradation of the water environment, as do continental-scale assessments in Europe (Stanners & Bourdeau, 1995; European Environment Agency, 1999). “Water” has been identified as the emerging critical environmental issue of the twenty-first century (Cosgrove &C Rijsberman, 2000; WMO, 1997).
The aim of this book is to explore the linkages between global environmental change and the hydrological system: it examines how changes in the catchment and the inputs to the catchment affect hydrological regimes, and also assesses the role played by hydrological processes in global environmental change. It focuses on the hydrological system at the land surface, rather than over the ocean. This introductory chapter places hydrology in the context of earth system science, introduces the concept of global environmental change, and describes the changing way in which hydrology is being studied.
1.2 Hydrology and earth system science
Hydrology is broadly defined as the study of the occurrence, distribution, circulation and properties of water on the earth, and the hydrological cycle (Figure 1.1) lies at the heart of hydrological science. All the water that falls as precipitation has evaporated from the land and the oceans. Rivers transport some of the precipitated water across the land surface, to be evaporated elsewhere - from the sea or a lake, for example - and much is transported from one place to another as water vapour in the atmosphere. Water is being continuously recycled through the oceans, atmosphere, lithosphere, cryosphere (ice sheets, glaciers and permafrost) and biosphere (vegetation). Hydrology is therefore central to any understanding of the way the earth system works. Hydrological science can be seen as the component of “geoscience” that links land, vegetation, atmosphere and ocean (Figure 1.2), and which is a part of earth system science. In practice, hydrologists are usually concerned with freshwater (oceanographers deal with the oceans) and with water once it reaches the land surface (meteorologists and atmospheric chemists deal with water when it is in the atmosphere). They have also traditionally taken a catchment approach, treating the inputs to the catchment of water and energy as given, and focusing on the translation of these inputs to “outputs” of streamflow. Increasingly, however, hydrologists are working with meteorologists to understand the way the land surface affects the atmosphere - and hence the “inputs” to the catchment - and with oceanographers to contribute to the understanding of the way in which flows of water and material to the sea affect coastal and ocean processes. Hydrologists are also working with plant physiologists to investigate the process of transpiration, and with ecologists to understand the hydrological controls on ecosystem characteristics.
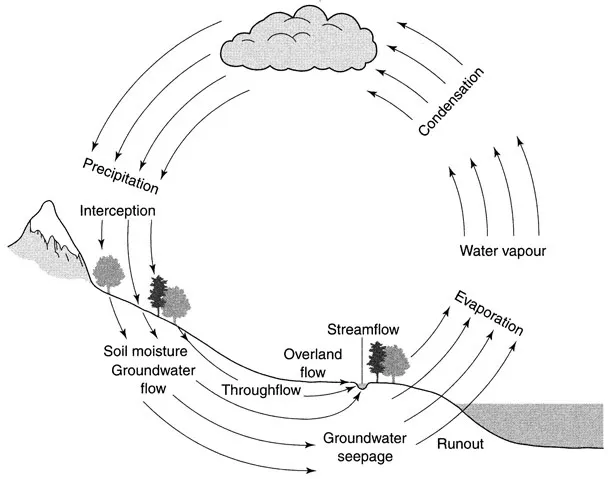
Figure 1.1 The hydrological cycle (Ward 8t Robinson, 2000)
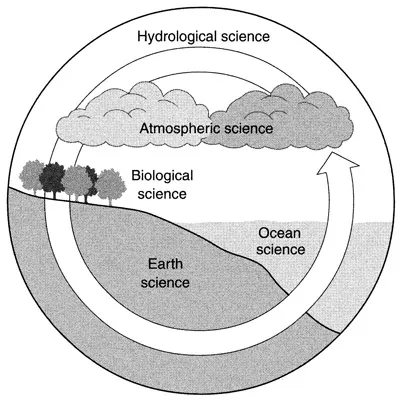
Figure 1.2 Hydrological science as a component of earth system science (modified from National Research Council, 1991)
Central to the concept of earth system science is the idea of cycles of energy and material. The hydrological cycle is an obvious example, but interest has developed over the past decade in biogeochemical cycles in general. A biogeo-chemical cycle can be defined as the cycle of any element or compound, through all its states and phase changes. Attention is often focused on the cycles of the key elements carbon, nitrogen, phosphorus, sulphur and oxygen, although it is of course possible to construct cycles for any flux of material - including sediment. Cycles can be evaluated at all scales, but only at the global scale are cycles closed with no inputs to or output from the system of interest. At smaller scales, such as the catchment or indeed continent, there will be inputs and outputs across the system boundary. Water plays a key role in all biogeo-chemical cycles, both as a means of transporting material from one store to another and as a medium in which transformations take place. One of water’s unique features is that virtually all matter can dissolve in it, and biological and chemical transformations can also occur in water to alter the speciation of elements. Any assessment of any biogeochemical cycle must therefore consider the role of hydrological processes.
1.3 Hydrology and global environmental change
The earth is over 4.5 billion years old, and over this entire period has been subject to change. Geological forces include such processes as plate tectonics, metamorphism and vulcanism, which shape the broad pattern of land surface and ocean. These are internal processes, essentially driven by heat at the earth’s core. Tectonic movement and metamorphism occur over time scales of millions of years, but the effects of vulcanism can be immediate and catastrophic. Three sets of external forces affect the earth system. The atmosphere, hydro-sphere and biosphere are all driven by inputs of energy from the sun, which varies diurnally as the earth rotates, seasonally as the earth revolves around the sun, and as solar output fluctuates (solar luminosity varies by ±0.07% over the 11-year sunspot cycle (Hoffert et al., 1988), and has varied more over much longer time scales). The gravitational interaction between the earth and the moon generates tides, which vary predictably from day to day, and gravitational interactions between the earth and other planets produce changes in the earth’s orbit over millennial time scales which alter the amount of solar energy received. Thirdly, meteorites sometimes hit the earth, with occasionally very significant consequences. Additional to these geological and external forces are rhythms and patterns inherent in the linked atmosphere-ocean system. These rhythms, which operate on annual or decadal time scales, arise in some cases because of the different rate of response of different parts of the atmosphere-ocean system to an external forcing, and in others because the system undergoes a step change once a small progressive change pushes the system beyond a critical internal threshold. The El Nino-Southern Oscillation is an example of such a rhythm.
Superimposed onto this “natural” global environmental change, which operates at all time scales, are the effects of various human activities. The cultivation of wheat and barley began in the Middle East around 10 000 years ago, and clustered settlements began to appear at around the same time; 7000 years ago farmers in the Indus Valley and Mesopotamia were irrigating crops. Humans have therefore been impacting upon their environment for many thousands of years. However, the rate of change has been accelerating dramatically, and Figure 1.3 shows changes in a number of indicators of human activity since the middle of the twentieth century. These interventions are affecting hydrological systems in many catchments.

Figure 1.3 Indicators of human intervention: population, agriculture, CO2 emissions and the exploitation of water between 1950 and 2000. Population and agriculture data from the FAO’s FAOSTAT database (http://apps.fao.org), CO2 emissions from fossil fuels from Marland et al (1998), CO2 emissions from land use change from Houghton (1999), water withdrawals from Shiklomanov (1998), and numbers of registered dams from the ICOLD database (www.icold.org). The CO2 emissions data are available from http://cdiac.esd.ornl.gov
As Turner et al. (1990) explain, there are two components of human-induced global environmental change.1 Systemic changes are operating at global scales. They include climate change due to the increasing concentration of greenhouse gases in the atmosphere, acid rain and depletion of stratospheric ozone. Cumulative change is the net effect of many different changes occurring at different spatial scales. Land cover change, for example, may be occurring in different places for many different reasons, although some of the economic drivers - such as globalisation - may be common. Figure 1.4 conceptualises the implications of global environmental change for the hydrological system. Changes in catchment land cover, the use of water in the catchment, and the development of physical infrastructure in the catchment (such as regulating reservoirs and flood embankments) can be seen as cumulative global environmental change. Changes to the inputs to the catchment - in terms of the amount of water, energy and dissolved material - are due to systemic global environmental change. These two types of change affect hydrological processes within the catchment, but also feed through the linked earth system to affect other catchments. Changes in soil moisture, for example, due to changes in land cover, will affect the amount of water available to be evaporated back up into the atmosphere, and hence rainfall downwind. Changes in the output of water and material from a catchment to the sea affect coastal and possibly deep ocean processes, potentially affecting regional and global climate. Global environmental change should not therefore be seen as just affecting the catchment: what happens in the catchment affects global environmental change.
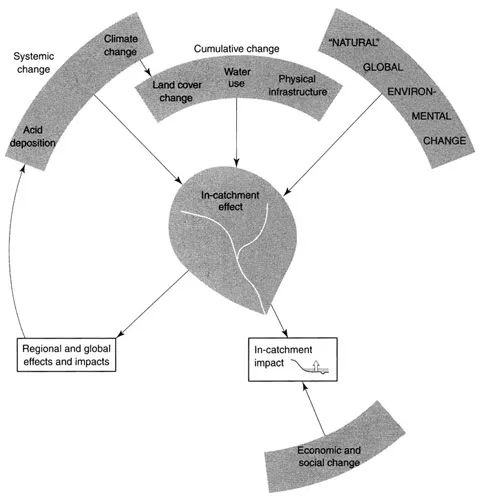
Figure 1.4 Global environmental change and the hydrological syste
Both natural and human-induced global environmental change affect the hydrological processes and regimes within a catchment. The impacts of these changes, however, depend on the characteristics of the human use of the catchment and the ecological characteristics of the water environment. Table 1.1 summarises some of the potential human and environmental impacts of hydrological change: the list is not exhaustive. Many of these impacts are affected not only by hydrological change, but also by economic and social changes altering the exposure to environmental hazard. In the broadest terms, “hazard” is a function of both the physical environment and the human environment: an increase in the impact of water-related hazards, such as floody is therefore not necessarily due to global environmental change altering the physical environment. It may be entirely due to increasing exposure to the hazard.
Table 1.1 Example impacts of adverse hydrological change in the catchment
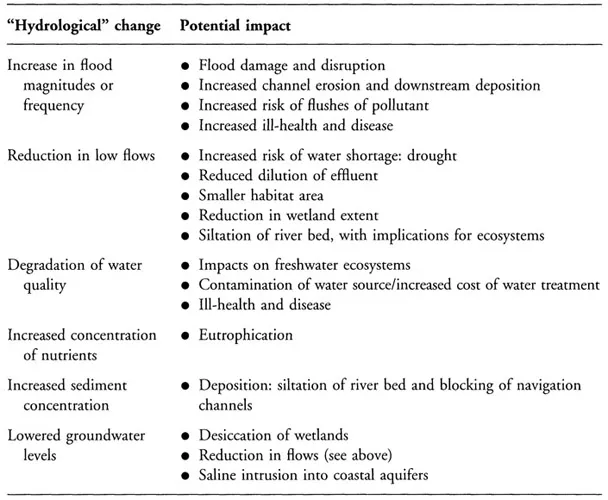
1.4 Hydrology in practice
Traditionally, hydrologists have taken a catchment perspective, seeing precipitation and energy as inputs and focusing on the characteristics of the output of streamflow. This largely reflects hydrology’s disciplinary tradition as an applied science, aimed at developing techniques to estimate hydrological characteristics at a site as part of the process of water management. Much hydrology has therefore concerned itself with issues such as flood frequency estimation and the stochastic generation of data, central to the sound design of engineering works, and until at least the late 1970s most was done by hydrologists who trained as engineers and who were working to solve engineering problems.
Since the early 1980s the centre of gravity of hydrology has shifted towards the geosciences, and ...
Table of contents
- Cover
- Half Title
- Title
- Copyright
- Dedication
- Contents
- Series preface
- Volume preface
- Acknowledgements
- Chapter 1 Hydrology, earth system science and global environmental change
- Chapter 2 The global water balance
- Chapter 3 Components of the water balance
- Chapter 4 Patterns of hydrological behaviour
- Chapter 5 Water quality and the flux of materials
- Chapter 6 Change in the catchment
- Chapter 7 Changes to the inputs to the catchment: acid deposition and global warming
- Chapter 8 Hydrological processes and the earth system
- Chapter 9 Hydrology and global environmental change: an overview
- Appendix 1 Acronyms
- Appendix 2 Web-sites
- References
- Index
- Plates