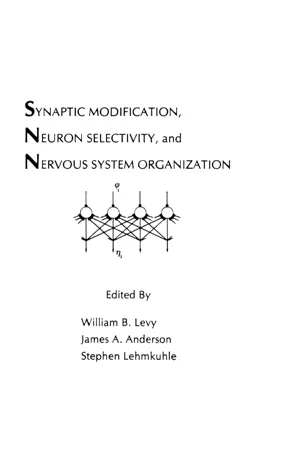
eBook - ePub
Synaptic Modification, Neuron Selectivity, and Nervous System Organization
This is a test
- 280 pages
- English
- ePUB (mobile friendly)
- Available on iOS & Android
eBook - ePub
Synaptic Modification, Neuron Selectivity, and Nervous System Organization
Book details
Book preview
Table of contents
Citations
About This Book
First published in 1985. This is a collection of essays presenting various theoretical ideas concerning distributive memories and cortical models. In addition, it includes a discussion of how a central set of assumptions can explain much of what is now known about deprivation and normal development of visual cortex and can perhaps also serve as the basis for a theory of higher nervous system organization as well as the Changeux model of how a synapse can modify itself.
Frequently asked questions
At the moment all of our mobile-responsive ePub books are available to download via the app. Most of our PDFs are also available to download and we're working on making the final remaining ones downloadable now. Learn more here.
Both plans give you full access to the library and all of Perlegoâs features. The only differences are the price and subscription period: With the annual plan youâll save around 30% compared to 12 months on the monthly plan.
We are an online textbook subscription service, where you can get access to an entire online library for less than the price of a single book per month. With over 1 million books across 1000+ topics, weâve got you covered! Learn more here.
Look out for the read-aloud symbol on your next book to see if you can listen to it. The read-aloud tool reads text aloud for you, highlighting the text as it is being read. You can pause it, speed it up and slow it down. Learn more here.
Yes, you can access Synaptic Modification, Neuron Selectivity, and Nervous System Organization by William B. Levy,James A. Anderson,Stephen Lehmkuhle in PDF and/or ePUB format, as well as other popular books in Psychology & History & Theory in Psychology. We have over one million books available in our catalogue for you to explore.
Information
1 | Associative Changes at the Synapse: LTP in the Hippocampus |
University of Virginia School of Medicine
One of our primary research goals is to define the rules governing the alteration of synapses. This chapter focuses on our studies of long-term potentiation (LTP) and synaptic modification following convergent, temporally associated neural activity.
The phenomena of LTP were first hinted at by Lømo in abstract form (Lømo, 1966) and were subsequently reported in detail by Bliss and Lømo (1973) and Bliss and Gardner-Medwin (1973). These studies report that delivering brief, high-frequency conditioning pulses to a monosynaptic system results in a long-lasting increase of the synaptic excitation by these afferents upon their postsynaptic cells. Bliss and Lømo argue, as do McNaughton, Douglas, and Goddard (1978) and ourselves (Levy & Steward, 1979), for the synaptic nature of the changes involved in long-term potentiation. McNaughton et al. (1978) and ourselves (1979) argue that LTP is a cooperative phenomenon involving coexcitation of neuronal inputs and outputs similar to a proposal by Hebb (1949). Furthermore, we also argue that, in tandem with this associative rule for potentiation, these same synapses are also governed by a rather specific rule for removing this potentiation (the so-called phenomenon of âdepotentiationâ).
Though in agreement with the research that preceded ours (cited above), this chapter draws mainly from our own data to argue for the synaptic nature of LTP. Further, it defines more precisely the rules governing associative synaptic modifications with the hope that eventually these rules may take a precise mathematical form. Before discussing this topic certain technical aspects of the system are presented, in particular the anatomical and electrophysiological characteristics of the entorhinal projection to the dentate gyrus. LTP in this system is then described in detail in order to adduce the synaptic nature of LTP as an associative form of synaptic modification.
Introduction to the EC-DG System
The studies reported here use the monosynaptic projection from the Layer II cells of the entorhinal cortex (EC) to the granule cells of the dentate gyrus (DG) of the hippocampus. The axons of the Layer II cells project through the lower levels of EC to enter the angular bundle. Via this fiber tract and the perforant pathway, the EC afferents travel over and through the hippocampus proper in order to reach their synaptic targets (i.e., granule cell dendrites) in the molecular layer of the dentate gyrus. In addition to this very large ipsilateral projection, a small, very sparse Layer II EC projection forms synapses in the contralateral molecular layer. The EC afferents are topographically related to the molecular layer of the DG in the sense that the most laterally placed Layer II cells project to the most distal aspects of the molecular layer, whereas cells located in progressively more medial positions across the EC project to progressively more proximal locations in the molecular layer.
Consistent with the monosynaptic nature of this system, a strong afferent volley initiated in the angular bundle (and maximized for the medial EC afferents) results in an ipsilateral DG response with latencies as short as 1.8 msec. (LTP is detected even at these shortest latencies following the proper conditioning paradigm; Levy, unpublished observations.)
In the experiments that follow, the responses are all measured extracellularly and are of two types, the population EPSP (pEPSP) and the population spike. Lømo has shown (1971) with intracellular recording that the monosynaptic response from the entorhinal cortex to the dentate gyrus is depolarizing and excitatory in that it fires the granule cells of the dentate gyrus. Further, these intracellular events of synaptic depolarization and cell firing are well correlated with the responses of the extracellular electrical field. After a powerful discrete stimulation of EC afferents, measurements from the region of active EC-DG synapses register an initial negative voltage (correlated with local excitatory synaptic activation), quickly interrupted by a brief, positive-going wave form (correlated with the active discharge of granule cell somata and axons). As the electrode is advanced from these dendritic regions into the granule cell layer the polarity of these two wave forms reverses. In the region of the granule cell somata, individual action potentials are detected that are in general temporal correspondence to the population spike (pop. spike) response. LTP of the pEPSP slope is equivalently quantified using either the positive- or the negative-going form of the pEPSP (Wilson, Levy, & Steward, 1981). Thus, the extracellular technique can measure both synaptic responses and cell firing.

FIG. 1.1 Long-term potentiation (LTP) in the normal ipsilateral temporodentate circuit. The population EPSP (left column) and population spike (right column) were simultaneously recorded. Each response and data point represents a computer average of four evoked potentials. A: sample averaged evoked potentials obtained immediately prior to (a), immediately following (b), and 28 min following (c) 400 Hz conditioning. The responses correspond to points a, b, and c respectively in B.B:LTP of test responses. The test stimulus was delivered at 30 V at a rate of 0.05 Hz. LTP was induced by replacing eight test pulses with 60-V, 20-msec, 400-Hz trains (â). The break between post-LTP points b and c represents an 8-min period during which the post-LTP inputâoutput function of C was recorded. C: pre-LTP and post-LTP stimulus intensity-response magnitude relationships (inputâoutput functions). (Reprinted from Wilson et al., 1979).
LTP
When high frequency trains are used to activate the EC afferents, there can be a long-lasting increase of the pEPSP and pop. spike subsequently evoked from these conditioned afferents. Figure 1.1 is an example of this LTP. After establishing a baseline to the test pulse (delivered 1/30 sec) a total of 64 pulses are delivered at 8, 400 Hz, 8 pulse trains (one train every 10 sec). Thirty seconds after these conditioning trains, testing is resumed at 1/30 sec. Following the response in time (Fig. 1.1B) reveals a maintained increase of both the pEPSP and the pop. spike. For a group of eight animals, Wilson et al. (1979) showed little difference in the measured LTP at 5 to 10 minutes and 15 to 20 minutes postconditioning. For healthy animals with stable, low noise background activity, my general impression is that if an animal shows potentiation at 5 minutes postconditioning there is a 95% likelihood that an increase will be present at 10 minutes, and if present at 10 minutes, there is a 95% likelihood that it will be present at 20 minutes, and so on. In several animals we have followed potentiation for as long as 12 hours before terminating the experiment. Other investigators have followed LTP for a day or more (Bliss & Gardner-Medwin, 1973). Thus, very long-lasting increases in synaptic efficacy can result from brief high-frequency conditioning trains.

FIG. 1.2 Temporal correspondence of the population spike with cell firing before and during LTP. Pre- and postconditioning responses from one normal (ipsilateral EC-DG system) and one unilateral EC lesioned and sprouted animal (proliferated contralateral EC-DG system) are illustrated. Each trace is the sum of 16 individual responses. Poststimulus time histograms of evoked cell firing (lower trace in each record) were generated by filtering (2â6 KHz bandpass), amplifying, and discriminating responses recorded from the granule cell layer (upper trace in each record). (Reprinted from Wilson, 1981b).

FIG. 1.3 This graph plots the integrated cell firing of the two histograms in the bottom of Fig. 1.2. When plotted in this fashion, it is quite evident that a substantial increase in cell firing has occurred as predicted by the increased population spike seen in Fig. 1.2.
It is hypothetically possible that an increase of extracellular current flow such as observed with LTP might result from hyperpolarization of the postsynaptic cellâs resting potential rather than increased excitatory synaptic activation. However, any such postulated hyperpolarization would cause decreased cell firing and a delay of the pop. spike latency for equivalent pEPSP before and after conditioning. Such results are not the case. Figures 1.2 and 1.3 show that the increased population spike does correctly indicate increased cell firing.Figure 1.4 shows that instead of being shifted to the right, as predicted by the hyperpolarization hypothesis, the inputâoutput curve relating each pEPSP size to its associated pop. spike size is actually shifted to the left. This shift indicates, if anything, that the granule cells are relatively less inhibited after conditioning (cf. Wilson et al., 1981, for a more complete discussion of this shift to the left). Finally, examination of pop. spike latency for equivalent pEPSPs before and after potentiation reveals no delay of this latency (Fig. 1.5). Therefore, none of the evidence is consistent with the hyperpolarization hypothesis. It is concluded that LTP is characterized by larger synaptic excitation, which causes more cell firing (additional evidence for the synaptic nature of LTP is found in the following section).
Associative Synaptic Activation and LTP
The work of McNaughton et al. (1978) begins the systematic investigation of conditioning stimulus intensity upon LTP. These researchers showed that high-frequency conditioning trains to the angular bundle did not evoke LTP unless the intensity surpassed an apparent minimum or critical intensity. In this type of experiment, a single intensity test pulse is used while conditioning pulse intensity is varied. The implication of this work is of preeminent importanceâthere is an apparent critical minimum number of coactive af-ferents required to induce LTP.

FIG. 1.4 Conditioning-induced changes in population spike/population EPSP relationships. Each group mean population spike is plotted as a function of the population EPSP that generated it. Preconditioning and 5-min postconditioning profiles are illustrated for both medium intensity (left side) and high intensity (right side) conditioning. An arrow (â) indicates the average population EPSP size evoked at the conditioning stimulus intensity in each plot. (Reprinted from Wilson, 1981b).

FIG. 1.5 Conditioning-induced changes in the relationship of the population spike and population EPSP to spike onset latency in normal animals. Onset latency was normalized in each animal as the percentage of the preconditioning range from longest (0%) to shortest (100%) latency. In A, the decrease in group mean population spike onset latency is plotted as a function of the population EPSP measure prior to and following high intensity conditioning. In B, population spike size is plotted as a function of the change in onset latency for the same train. An arrow (â) indicates the size of the population EPSP (A) or the decrease in onset latency (B) at the average conditioning intensity. (Reprinted from Wilson, 1981b).
In our laboratory Wilson has investigated this phenomenon in some detail. Based on comparisons from eight animals, he found LTP in only two animals when the stimulus intensity was adjusted below that giving a population spike. Increasing the conditioning intensity so that a small pop. spike was obtained resulted in potentiation for six of the eight animals. Finally, using a very powerful stimulus intensity that evoked a large population spike, he was able to demonstrate potentiation in s...
Table of contents
- Cover
- Half Title
- Title Page
- Copyright Page
- Table of Contents
- Introductory Remarks
- Chapter 1 Associative Changes at the Synapse: LTP in the Hippocampus
- Chapter 2 Hebbian Modification of Synaptic Transmission as a Common Mechanism in Experience-Dependent Maturation of Cortical Functions
- Chapter 3 Mondayâs Discussion
- Chapter 4 Some Constraints Operating on the Synaptic Modifications Underlying Binocular Competition in the Developing Visual Cortex
- Chapter 5 Remarks About the âSingularityâ of Nerve Cells and its Ontogenesis
- Chapter 6 The Rules of Elemental Synaptic Plasticity
- Chapter 7 Neural Problem Solving
- Chapter 8 What Hebb Synapses Build
- Chapter 9 Neuron Selectivity: Single Neuron and Neuron Networks
- Chapter 10 Tuesdayâs Discussion
- Chapter 11 A Model of Cortical Associative Memory
- Chapter 12 What Do Drug-Induced Visual Hallucinations Tell Us About the Brain?
- Chapter 13 Wednesdayâs Discussion
- Author Index
- Subject Index