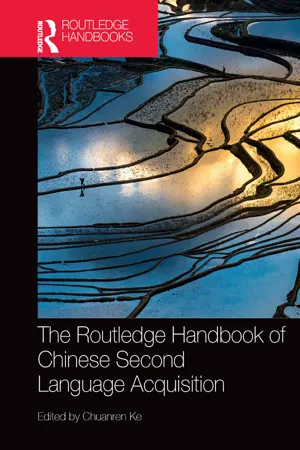
This is a test
- 459 pages
- English
- ePUB (mobile friendly)
- Available on iOS & Android
eBook - ePub
The Routledge Handbook of Chinese Second Language Acquisition
Book details
Book preview
Table of contents
Citations
About This Book
The Routledge Handbook of Chinese Second Language Acquisition is the first reference work of its kind.
The handbook contains twenty contributions from leading experts in the field of Chinese SLA, covering a wide range of topics such as social contexts, linguistic perspectives, skill learning, individual differences and learning settings and testing.
Each chapter covers historical perspectives, core issues and key findings, research approaches, pedagogical implications, future research direction and additional references.
The Routledge Handbook of Chinese Second Language Acquisition is an essential reference for Chinese language teachers and researchers in Chinese applied linguistics and second language acquisition.
Frequently asked questions
At the moment all of our mobile-responsive ePub books are available to download via the app. Most of our PDFs are also available to download and we're working on making the final remaining ones downloadable now. Learn more here.
Both plans give you full access to the library and all of Perlegoâs features. The only differences are the price and subscription period: With the annual plan youâll save around 30% compared to 12 months on the monthly plan.
We are an online textbook subscription service, where you can get access to an entire online library for less than the price of a single book per month. With over 1 million books across 1000+ topics, weâve got you covered! Learn more here.
Look out for the read-aloud symbol on your next book to see if you can listen to it. The read-aloud tool reads text aloud for you, highlighting the text as it is being read. You can pause it, speed it up and slow it down. Learn more here.
Yes, you can access The Routledge Handbook of Chinese Second Language Acquisition by Chuanren Ke, Chuanren Ke in PDF and/or ePUB format, as well as other popular books in Languages & Linguistics & Languages. We have over one million books available in our catalogue for you to explore.
Information
Part I
Theoretical and methodological approaches to the study of second language Chinese
1
Neurocognitive approaches to Chinese second language learning1
Historical perspectives
In todayâs globally connected world, more people are learning a second language (henceforth L2) than at any other time before. But for most adults, learning a second language is a life experience that requires significant effort and time. The study of second language learning has important practical applications, but it also helps to address fundamental scientific questions (which will be the goal of the current chapter). For example, what happens to the learnerâs brain during and after learning a second language? Can learning of a new language modify neuroanatomy of the brain? What are the neurocognitive benefits of learning a second language, if any? Why are some people better and faster than others at learning a second language? These are some of the questions that have not received clear answers today, despite significant progress so far in the cognitive science of bilingualism (see Dong & Li, 2015; Li, 2015 for reviews).
Since the mid-to-late 1990s, a large number of neurocognitive studies, using neuroimaging methods such as functional magnetic resonance imaging (fMRI), positron emission tomography (PET), and electroencephalography/event-related potentials (EEG/ERPs), have revealed specific functional brain patterns in the learning of a second language (L2) (see reviews in Abutalebi, Cappa, & Perani, 2005; Costa & Sebastiån-Gallés, 2014; Hernandez, 2013; Indefrey, 2006; Li & Tokowicz, 2011; Van Hell & Tokowicz, 2010). These studies indicate that in contrast to predictions of the critical period hypothesis (Lenneberg, 1967), L2 learning, even if it occurs late in adulthood, leads to both behavioral and neural changes that may approximate the patterns of native or first language (L1).
Brain imaging studies on individual differences and second language learning revealed significant differences between successful learners and less successful learners in terms of their brain activation in left superior temporal gyrus before and after training (e.g., Wong, Perrachione, & Parrish, 2007). Breitenstein et al. (2005) explored brain regions associated with novel word learning. The authors used the learnersâ behavioral performance on a subsequent test of lexical knowledge as a measure of their vocabulary proficiency and correlated the proficiency level with their brain functional changes associated with learning. They found that the increase in novel word proficiency over the 50-minute training session was paralleled by a linear decrease of fMRI signal changes in the left hippocampus. Therefore they claimed that learning-related hippocampal activity is a stable marker of individual differences in the ability to acquire new vocabularies.
Recent interests in brain connectivity and large-scale brain networks point to a new direction for understanding not just activations of individual brain regions, but also the spatial and temporal relationships between multiple brain regions during cognitive and linguistic processing (Bressler & Menon, 2010; Poldrack, 2012; Sporns, 2010). This represents the view of cognitive functions as arising from the interactions between and within distributed brain systems, often constrained by context and mode of learning and processing. Within this perspective, a number of recent studies have examined L2 vocabulary learning with the fMRI methods. For example, Saidi et al. (2013) tested 12 Persian adults who had received intensive computerized French lexical training and collected fMRI data during a picture naming task at two time points: shallow learning phase (one week after training, mean accuracy rate = 69.9%) and consolidation learning phase (30 days after training, mean accuracy rate = 89.74%). Their results showed that language proficiency modulates functional integration levels within contributing circuits in L2 vocabulary learning.
Even more surprising is that the neural patterns of L2 experience are often, if not always, accompanied by anatomical changes in brain structure. Aided by methods of structural magnetic resonance imaging (sMRI) and diffusion tensor imaging (DTI), anatomical changes can be revealed as changes in gray matter (GM) density, cortical thickness (CT), or white matter (WM) integrity. A number of recent studies indicate that second language experience-induced brain changes including increased GM density and WM integrity can be found in children, young adults, and the elderly, and that these anatomical changes similar with the functional changes are sensitive to age, age of acquisition (AoA), L2 proficiency, language-specific characteristics, and individual differences (Elmer, HÀnggi, & JÀncke, 2014; Hosoda, Tanaka, Nariai, Honda, & Hanakawa, 2013; MrÄtensson et al., 2012; Schlegel, Rudelson, & Peter, 2012; Stein et al., 2012; Wong et al., 2008; see Li, Legault, & Litcofsky, 2014 for reviews).
Although the majority of the neuroscience/neuroimaging studies of second language learning have focused on Indo-European or other Western languages, an increasing number of studies have begun to examine Asian or Chinese languages in the second language or bilingual learning contexts (see two specific issues edited by Li & Green, 2007; Tan & Li, 2015). These studies have examined the neurocognitive differences between L1 and L2 of bilinguals in terms of language representation and processing, and how the similarities and differences can be modulated by learner-internal and external variables such as L2 AoA and L2 proficiency (Abutalebi, 2008; Hernandez & Li, 2007; Indefrey, 2006; Kotz, 2009; Morgan-Short, Faretta-Stutenberg, & Bartlett-Hsu, 2015; Mueller, 2005; Perani et al., 1998; Van Hell & Tokowicz, 2010; Weber et al., 2013). This chapter focuses on how this interdisciplinary approach is applied to L2 learning both in general and in the Chinese context. However, before we discuss further, we provide a background introduction to most common neuroimaging techniques, typical research paradigms, and brain network analyses in neuroimaging studies of Chinese second language learning.
Research approaches
Neuroimaging techniques
Thanks to the recent advances in neuroscience, we can explore the function and structure of the brain using a variety of different behavioral and brain measures. Consistent with this chapterâs focus on brain-based approaches, here we provide a short overview of four most commonly used measures: the hemodynamic neuroimaging approach of fMRI, the electrophysiological technique of ERP, sMRI for gray matter changes and DTI for tracking white matter fiber structure. Note that other methods, such as the lesion method, magnetoencephalagraphy (MEG), direct cortical brain stimulation, and transcranial magnetic stimulation (TMS), have also been used in L2 research but are beyond the scope of this chapter.
Functional magnetic resonance imaging (fMRI). FMRI involves the use of strong magnetic fields created by the magnetic coils of the MRI machine to measure hemodynamic changes in blood flow, specifically the blood-oxygen-level-dependent (BOLD) signals, a ratio of oxygenated versus deoxygenated hemoglobin in given brain regions. As we engage in cognitive and linguistic behavior, neuronal cells in certain brain regions consume more energy than in others, and the energy is supplied by hemoglobins, the red proteins that transport oxygen through the red blood cells. FMRI captures these dynamic BOLD activities in various parts of the brain, presumably reflecting underlying neuronal activities related to specific processes of cognition. In general, increased BOLD signals reflect increased cognitive activities, and by comparing the different BOLD signals from cognitive tasks versus those from a baseline task (e.g., a task in which the participant stares at a crosshair on a computer screen), fMRI researchers can make inferences about the role that focused brain regions play in a specific task, be it face recognition or language processing. The spatial resolution of fMRI is excellent by todayâs standard, in millimeter range (e.g., an fMRI voxel may be 1â4 cubic mm in size).
Event-related potentials (ERPs). This method measures the brainâs ongoing electrical activities on a millisecond-by-millisecond basis, a time window within which critical cognitive and linguistic processes take place. When raw EEG signals are averaged over multiple trials of a stimulus condition, and when these signals are âtime-lockedâ to stimulus events (e.g., presentation of a visual word), the corresponding âevent-relatedâ potentials that fluctuate in voltage can be extracted and analyzed. These fluctuations are designated as âcomponentsâ, the âbrainwave peaks and valleysâ. ERP components vary in a number of dimensions, including polarity (positive vs. negative), latency (timing), and amplitude (level), along with distribution information (location) of the activation on the scalp. These components are typically labeled according to the latency of the waveformâs peak amplitude; for example, N400 refers to a negative going waveform peaking at about 400ms post-stimulus onset during visual or auditory presentation. Several key components implicated in language processing have been identified in the literature (see Kutas, Federmeier, Staab, & Kluender, 2007 for a review) including N400, indicating lexical semantic integration in sentences, LAN (left anterior negativity, occurring in the same time window as N400), indicating morpho-syntactic analysis, and P600, indicating syntactic analysis and repair (see Key, Dove, & Maguire, 2005 for a summary of major ERP components relevant to cognition).
Structural magnetic resonance imaging (sMRI). It provides information to qualitatively and quantitatively describe the shape, size, and integrity of gray and white matter structures in the brain. Broadly speaking, MRI signal varies across tissue types because gray matter contains more cell bodies (e.g., neurons and glial cells) than white matter, which is primarily composed of long-range nerve fibers (myelinated axons), along with supporting glial cells. GM density or volume, as one of the most common measures of anatomical brain changes, can be calculated via voxel-based morphometry (VBM), an analytic method that extracts GM information from sMRI scans (see Ashburner & Friston, 2000; Mechelli, Price, Friston, & Ashburner, 2005). VBM typically involves the normalization of each brain scan to a standard stereotactic space (e.g., MNI space), delineation of GM versus WM versus cerebrospinal fluid (CSF), and a voxel-by-voxel analysis of the tissue concentration. VBM identifies the local tissue environment after correction for macroscopic anatomical differences across participants. Besides GM density, sMRI scans can also provide information on cortical thickness (CT) (Fischl & Dale, 2000; Im et al., 2008; Lerch & Evans, 2005). Unlike GM density or volume, CT is a direct measure of cortical morphology. In this technique, voxels are first segmented into GM, WM, or CSF. The boundaries between GM and WM, and between GM and CSF are then delineated either manually or through automated procedures. Finally, the thickness between these surfaces is measured using a variety of methods, each determining the distance between nodes on each surface for the entirety of the cortex examined. CT provides sub-millimeter accuracy and takes into account the folding of the cortical surface. Structurally there may be an inverse relationship between CT and GM due to the cortical folding patterns: thicker cortical regions are less convoluted and therefore have less GM density (see Chung, Dalton, Shen, Evans, & Davidson, 2006).
Diffusion tensor imaging (DTI). It is a MRI-based neuroimaging technique which makes it possible to visualize the location, orientation, and anisotropy of the brainâs white matter tracts. DTI examines the diffusion of water molecules in the brain and compares the degree of diffusivity of neurons along the axon, referred to as axial diffusivity (AD) along with the radial diffusivity (RD) that is perpendicular to the axon diameter (Filler, 2009). Another measure, the mean diffusivity (MD), is used to measure diffusion within a voxel, regardless of orientation, and is calculated by averaging the eigenvalues (Alexander, Lee, Lazar, & Field, 2007). Lower MD values often correspond to greater WM integrity. By far the most commonly used value to calculate the magnitude of diffusion is the fractional anisotropy (FA), a normalized standard diffusivity value between 0 and 1 calculated from the eigenvectors of the diffusion tensor (Assaf & Pasternak, 2008). The higher the FA value, the more integrity the WM has (contrasting the interpretation of the MD value). In studies of English and other Western languages, two WM tracts have been most strongly associated with language representation: the left arcuate fasciculus (AF) component of the superior longitudinal fasciculus (SLF) and the left inferior longitudinal fasciculus (ILF). First, the SLF connects a dorsal language network (Hickok & Poeppel, 2004, 2007), and may consist of (1) a direct AF pathway connecting posterior (superior temporal gyrus/Wernickeâs area) and anterior (inferior frontal gyrus/Brocaâs area) language cortical regions and (2) an indirect pathway including the SLF connecting t...
Table of contents
- Cover
- Half Title
- Title Page
- Copyright Page
- Table of Contents
- List of tables
- List of contributors
- Chinese SLA: introduction and future directions
- Part I Theoretical and methodological approaches to the study of second language Chinese
- Part II L2 Chinese skills development
- Part III Sociocontextual factors and individual differences in L2 Chinese development
- Part IV Classroom instruction and technology
- Subject index
- Author index