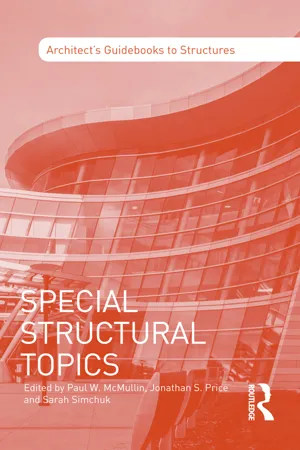
eBook - ePub
Special Structural Topics
This is a test
- 284 pages
- English
- ePUB (mobile friendly)
- Available on iOS & Android
eBook - ePub
Special Structural Topics
Book details
Book preview
Table of contents
Citations
About This Book
Special Structural Topics covers specialty structural situations for students and professional architects and engineers, such as soil mechanics, structural retrofit, structural integrity, cladding design, blast considerations, vibration, and structural sustainability. As part of the Architect's Guidebooks to Structures series, it provides a comprehensive overview using both imperial and metric units of measurement with more than 150 images. As a compact summary of key ideas, it is ideal for anyone needing a quick guide to specialty structural considerations.
Frequently asked questions
At the moment all of our mobile-responsive ePub books are available to download via the app. Most of our PDFs are also available to download and we're working on making the final remaining ones downloadable now. Learn more here.
Both plans give you full access to the library and all of Perlegoâs features. The only differences are the price and subscription period: With the annual plan youâll save around 30% compared to 12 months on the monthly plan.
We are an online textbook subscription service, where you can get access to an entire online library for less than the price of a single book per month. With over 1 million books across 1000+ topics, weâve got you covered! Learn more here.
Look out for the read-aloud symbol on your next book to see if you can listen to it. The read-aloud tool reads text aloud for you, highlighting the text as it is being read. You can pause it, speed it up and slow it down. Learn more here.
Yes, you can access Special Structural Topics by Paul McMullin, Jonathan Price, Sarah Simchuk, Paul W. McMullin, Jonathan S. Price, Sarah Simchuk in PDF and/or ePUB format, as well as other popular books in Architecture & Architecture Methods & Materials. We have over one million books available in our catalogue for you to explore.
Information
Chapter 1
Vibration
1.1 Theory
1.2 Vibration Causes
1.3 Vibration Design
1.4 Advanced Technology
1.5 Summary
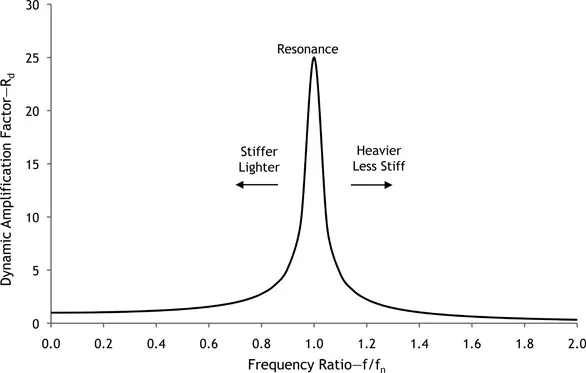
Figure 1.1 Dynamic Amplification Factor
Vibration problems can be vexing at best, and life-threatening at worst. They can seem like a serious mystery if we donât have the right analytical and experimental tools. With a grasp of basic dynamic principles, we can understand the office floor that bounces every time someone walks by, or the large rotating machine that seems to be bringing down the house.
Unlike strength or deflection design, where we want to be under the maximum permitted load (away from failure), safe vibration design is on either side of resonance, illustrated in Figure 1.1. This is where much of the challenge comes from. A change in mass or stiffness may shift us towards or away from resonance, depending on where we started. Vibration theory allows us to understand how variation of the input changes the structural response.
1.1 Theory
1.1.1 Load Types
You are familiar with static loads: dead, live, or snow. They vary slowly with time. Dynamic loads, on the other hand, change rapidly and are repetitive or transient in nature. We divide dynamic loads into periodic and non-periodic loads. Periodic loads are harmonic, often sinusoidal, loads. A rotating machine or a rhythmic human activity such as aerobics is an example of a harmonic load. Non-periodic loads may be impact loads or bomb blasts.
In this chapter, we will be focusing on harmonic loads caused by rotating machinery and human activity. Letâs begin with a discussion of key dynamics concepts.
1.1.2 Mode Shapes
Structures, such as strings, vibrate at different frequencies based on material and geometric stiffness. Each fundamental frequency of vibration is known as a mode. If we take the string in Figure 1.2, we see it vibrates in a parabolic shape from end to end (mode 1), from end to midpoint (mode 2), and in thirds (mode 3).
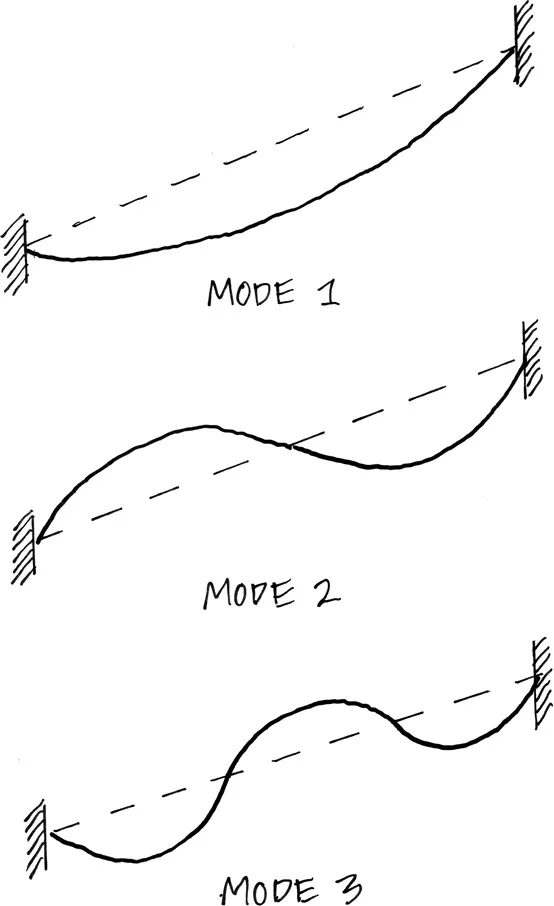
Figure 1.2 Mode Shapes of a String
Each mode shows us a different vibration shape. Figure 1.3 shows a mode shape associated with one of the fundamental vertical natural frequencies for a simple steel structure, in which we see hints of the vibrating string. Diagrams of the deflected shape help us visualize what is happening when we are designing for vibration.
Generally, we are concerned about the lower modes; however, depending on structuresâ mass and stiffness distribution, the higher modes can become important as well.1
1.1.3 Frequency
Frequency is a measure of vibration rate. We measure it in Hertz (Hz, cycles per second) or revolutions per minute (rpm). We concern ourselves with forcing and natural frequencies. Equipment rotation speed and human activities are examples of forcing (exciting) frequencyâthey drive the problem. Natural frequency represents the rate at which a structure vibrates if there are no continuous external forces present on a structure2âthey determine response to the input.
Mathematically, natural frequency is defined as:
(1.1) |
where:
- fn = natural frequency of the structure, Hz
- k = stiffness of the structure, lb/in (N/m)
- m = mass of the structure, lb (kg)
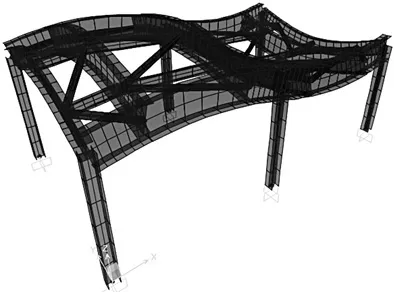
Figure 1.3 A Mode Shape of a Simple Steel Structure
Or, it can be defined as:
(1.2) |
where:
- g = acceleration of gravity, 386.4 in/s2 (9.81 m/s2)
- δ = static deflection, in (mm)
Natural frequency is a function of stiffness and mass. The more material, the greater the stiffness, the greater the frequency. The more mass, the lower the frequency.
Equation (1.2) will be more familiar to structural engineers, who are accustomed to calculating deflections. It also captures the compound effects of multiple deflection sources, such as girders and columns.
Figure 1.4 shows the frequency solutions for beams with different end conditions. These simple frequency solutions provide a starting point for vibration analysis, or for checking complex software solutions.
1.1.4 Resonance
When the forcing and natural frequencies get close to each other, resonance occurs. The result of being close to resonance may only be annoying, such as a floor vibration, but it also may cause a machine to malfunction, or lead to complete structural collapse.
The Tacoma Narrows Bridge is a good example of the forcing and natural frequencies getting too close. Tacoma Narrows Bridge was a suspension bridge with a total length of 5,939 ft (1,810 m). Called Galloping Gertie, the bridge vibrated vertically when the wind was blowing around 42 mph (67.6...
Table of contents
- Cover
- Title
- Copyright
- Dedication
- Contents
- Acknowledgments
- List of Contributors
- Introduction
- 1 Vibration
- 2 Blast Design
- 3 Sustainable Structures
- 4 Performance-Based Seismic Design
- 5 Structural Retrofit
- 6 Geotechnical Considerations
- 7 Structural Integrity
- 8 Cladding
- 9 Fire Design
- 10 Quality and Inspection
- Appendix 1: List of Units
- Appendix 2: List of Symbols
- Appendix 3: Imperial and Metric Conversion Tables
- Glossary
- Bibliography
- Index