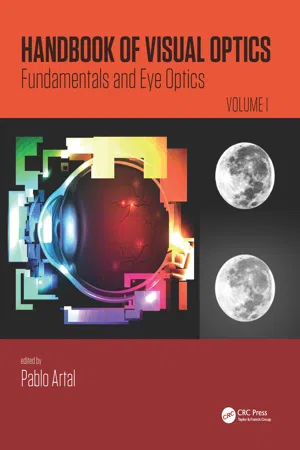
This is a test
- 437 pages
- English
- ePUB (mobile friendly)
- Available on iOS & Android
eBook - ePub
Book details
Book preview
Table of contents
Citations
About This Book
Handbook of Visual Optics offers an authoritative overview of encyclopedic knowledge in the field of physiological optics. It builds from fundamental concepts to the science and technology of instruments and practical procedures of vision correction, integrating expert knowledge from physics, medicine, biology, psychology, and engineering. The chapters comprehensively cover all aspects of modern study and practice, from optical principles and optics of the eye and retina to novel ophthalmic tools for imaging and visual testing, devices and techniques for visual correction, and the relationship between ocular optics and visual perception.
Frequently asked questions
At the moment all of our mobile-responsive ePub books are available to download via the app. Most of our PDFs are also available to download and we're working on making the final remaining ones downloadable now. Learn more here.
Both plans give you full access to the library and all of Perlegoâs features. The only differences are the price and subscription period: With the annual plan youâll save around 30% compared to 12 months on the monthly plan.
We are an online textbook subscription service, where you can get access to an entire online library for less than the price of a single book per month. With over 1 million books across 1000+ topics, weâve got you covered! Learn more here.
Look out for the read-aloud symbol on your next book to see if you can listen to it. The read-aloud tool reads text aloud for you, highlighting the text as it is being read. You can pause it, speed it up and slow it down. Learn more here.
Yes, you can access Handbook of Visual Optics, Volume One by Pablo Artal in PDF and/or ePUB format, as well as other popular books in Medicine & Surgery & Surgical Medicine. We have over one million books available in our catalogue for you to explore.
Information
Part I
Introduction
1 History of physiological optics in the twentieth century
Contents
1.1 Status at the beginning of the century
1.2 The foundations
1.3 Structural optics of the eye
1.3.1 Eye dimension and axial length
1.3.2 Cornea
1.3.3 The crystalline lens
1.3.4 Transmission of the ocular media
1.3.5 Retinal optics
1.4 The retinal image
1.4.1 Aberrations of the eye
1.4.2 Quality of the retinal image
1.4.3 Optical transfer function
1.4.4 Strehl ratio
1.4.5 Stray light
1.5 Ophthalmic instrumentation
1.5.1 Ophthalmoscopy
1.5.2 Optometers and automatic objective refractometers
1.6 Spurt at the end of the twentieth century
References
1.1 STATUS AT THE BEGINNING OF THE CENTURY
Physiological Optics, as confirmed by its central manifestation, Helmholtzâs three-volume handbook, was understood at the time to be synonymous with the current Vision Science. But nomenclature has to go along with the explosive expansion of scientific knowledge. Hence the more optical components are now subsumed under Visual Optics, and even here further subdivision is needed. Optical imagery in the living eye is continually conditioned on factors arising from being embedded in motor apparatuses, specifically those controlling the pupil aperture and the ciliary muscle. Hence a division into structural visual optics, relating to the image-forming properties of the static normal eye, and functional visual optics, which would fold in accommodative and aperture size factors, seems indicated. Though it is not recognized as a distinct discipline, one can identify a branch of research as histological optics. Insofar as it transmits light unimpeded, eye tissue, such as the cornea and the crystalline lens, needs to have unusual biological structure. This became more evident and constituted a challenge around the middle of the century when electron microscopy began to reveal the subcellular makeup of corneal and lenticular layers. The pioneering study by David Maurice (1957) on the cornea was influential here.
The final biological stage of light capture resides in the receptors. Starting with an observation by Ernst BrĂźcke in the 1840s, there have been consistent attempts to assign to them special light-gathering properties. The directional sensitivity and wave guide nature of retinal receptors has been an active area now for the last 80 years. It may be noted parenthetically that the optics of invertebrate eyes, left out of consideration in this review, has deservedly been given much attention (Exner 1891, Snyder and Menzel 1975, Land and Nilsson 2002).
1.2 THE FOUNDATIONS
The eyeâs image-forming properties were well understood in the middle of the eighteenth century as shown in the classic treatise by Robert Smith (1689â1768) (Smith 1738). Through the efforts of astronomers, for example, Bessel, Seidel, and Airy (1801â1892), optics as a discipline was thoroughly established in the nineteenth century. Maxwellâs (1831â1879) electromagnetic theory took command of the subject in 1861 and has never needed superseding. The giants of physiological optics, Thomas Young (1773â1839), Jan Purkinje (1787â1869), Listing (1808â1882), and Helmholtz (1821â1894), laid and cemented the foundations, and ophthalmologists Donders (1818â1889), Landolt (1846â1926), and Snellen (1834â1908) developed clinical applications. Thus, a century ago, at the time of the beginning of the First World War, a student had available comprehensive compendia containing the available knowledge, specifically Helmholtzâs Physiological Optics in the new edition updated in particular by Gullstrand (1862â1930), the Graefe-Saemisch Handbuch der Augenheilkunde in its many volumes and several editions, followed in the next couple of decades by important chapters in Vol XII of Bethe et al., Handbuch der normalen und pathologischen Physiologie (1932) and in Abderhaldenâs Handbuch (1920), and in Vol 1 of Duke-Elderâs Textbook of Ophthalmology (1932). When the author entered optometry school in 1940, the assigned textbook, the second edition of Emsleyâs Visual Optics (Emsley 1939), contained a treatment of the subject that would rival current accounts and in some respects exceed their scope. By that time, too, quantum theory insofar as its characterization of the photon was concerned had solid footing and quite soon thereafter gripped the vision community when used to underpin our understanding of the absolute visual threshold (Hecht et al. 1942). The vision community has been well served by the authoritative treatment of the subject by Yves LeGrand (1908â1986) in various versions of his textbook (LeGrand 1949), a model of clarity.
Exhaustive literature surveys of vision science up to the beginning of the twentieth century had been provided in the encyclopedic scholarship displayed in the appendixes by A. Koenig to the second edition of Helmholtzâs Physiological Optics (almost 8000 references up to 1894) and F. Hofmann in his Graefe-Saemisch Handbuch chapters (almost 1500 references on spatial vision alone) and by A. Tschermak in the voluminous footnotes of his chapters in Bethe et al. Handbuch (Tschermak 1931).
As will be seen, developments in visual optics during the second half of the twentieth century required an expanded view beyond geometrical optics and the simple application of diffraction theory in Airyâs disk, yet the foundations for it were well in place. Abbe (1873) and Rayleigh (1896) in their treatment of microscope resolution used a framework that contained, almost explicitly, all the elements that were to become mainstream in the Fourier theory of optics that has since become dominant. Though it was more of academic than practical interest at the time, the theory applicable to coherent light (and what is more challenging, partially coherent light) was put on the table by van Cittert (1934), and so were the celebrated polynomials of Zernike (1934).
The upswing in the growth of optics, specifically as it plays a role in vision, in the middle of the twentieth century thus had their origin elsewhere. Most prominently, it was the harnessing of scientific and industrial resources in the conduct of the Second World War that ended in 1945. The scientific community virtually unanimously rallied behind the war effort, contributing insight and inventiveness to a heady mixture that also included technological innovation and industrial prowess. After the war, this continued in university and some corporate laboratories, blossoming into a research enterprise of unprecedented magnitude and productivity. The sequence into cybernetics (Wiener 1948), information theory (Shannon and Weaver 1949), and the linear systems approach (Trimmer 1950) and control theory was seamless and so was the progress toward labs equipped with oscilloscopes, photomultipliers, digital computers, transistor devices, in time followed by lasers, LCD, and deformable mirrors. For decades, vision laboratories thrived on âwar surplusâ lenses, mirrors, prisms, and filters. Light that was once generated by candles, and had its intensity controlled by the inverse square law and its duration by episcotister disks, was produced by lamps with specific filaments and then by high-pressure mercury arcs with wavelength range restricted by narrowband interference filters and intensity adjusted by neutral density wedges. To achieve high retinal illuminance, the filaments were imaged in the pupil in Maxwellian view well before its optical subtleties had been realized (Westheimer 1966).
1.3 STRUCTURAL OPTICS OF THE EYE
1.3.1 EYE DIMENSION AND AXIAL LENGTH
The ingenious method of measuring the eyeâs axial length by utilizing x-ray phosphenes (Goldmann and Hagen 1942) soon gave way to sonography that in the form of corneal pachometry (Molinari 1982) is in clinical use and now has become a reliable means of evaluating the refractive needs associated with cataract extraction (Hoffer 1981).
1.3.2 CORNEA
Because it is the principal source of the eyeâs refractive power and because, unlike the other refractive surfaces, it is immediately accessible, the cornea has always attracted much attention. Gullstrand in his appendix to the Third Edition of Helmholtz went into much detail about the shape of the corneal surface and the various means of measuring it. Keratoscopy and keratometry formed a strong chapter in Abderhaldenâs Handbuch. As contact lenses became ubiquitous and their fitting needed good information of the corneal surface on which they rested and whose optical properties they largely preempted, rapid and accurate measurements of corneal curvature could be performed in the clinic by cleverly designed electro-optical apparatuses, the subject of continued attention and technical innovation (Fowler and Dave 1994). Polarization effects, which can be made visible, have been ascribed to the cornea (Stanworth and Naylor 1950).
1.3.3 THE CRYSTALLINE LENS
The anterior and posterior surfaces of the crystalline lens are of critical relevance in how the eye accommodates, that is, changes focus under neural control by contraction of the ciliary muscle. E.F. Fincham (1937a), in part using optical means, made the major contribution to this topic in his monograph. It became clear early in the optical modeling of the eye that the anterior and posterior curvature of the lens could not fully account for its total refractive power for a biologically realistic refractive index, and giving the eye a solid interior core was not supported by evidence. Hence the proposition that a remarkable proportion of its total refractive power is provided by a refractive index gradient had gained acceptance by the time Gullstrand wrote his 1911 appendix. More recent approaches show just how challenging a topic this is (Campbell 1984, Pierscionek and Chan 1989).
1.3.4 TRANSMISSION OF THE OCULAR MEDIA
For a variety of reasons, the transmissivity of the ocular media has been of interest, more recently because of concern for possible damage from exposure to intense sources. For decades the data accepted as authoritative came from the study by Ludvigh and McCarthy (1938). It formed the basis for the confirmation that the energy exchange at absolute visual threshold involved only a handful of photons (Hecht et al. 1942). Wavelength dependency of light absorption in the media became an issue in the characterization of retinal photopigments, one of the most important research enterprises of vision science in the middle of the twentieth century (Rushton 1959, Wald 1964).
1.3.5 RETINAL OPTICS
In the vertebrate, before it reaches the receptors, light has to traverse several retinal layers that therefore have to be essentially transparent, not necessarily a quality automatically associated with active neural tissue. Haidingerâs brushes, an entoptic phenomenon, have their origin in retinal optical structure (Naylor and Stanworth 1954). Myelination of the ganglion cell axons, helpful in enhancing velocity of action potentials, does not start till they exit the eyeball at the optic disk. The vascular tree of Purkinje, a prominent feature of the fundus, is somehow compensated for and made visible only by special tricks. The central region of the retina in the primate is suffused by a pigment, selectively absorbing light of some wavelengths. It seems to have a role in Haidingerâs brushes (Bone and Landrun 1984, Mission 1993), but whether it is the origin of the entoptic phenomenon known as Maxwellâs spot (Maxwell 1890/1965, p. 278) has been subject of an interesting debate (Polyak 1941, Walls and Mathews 1952). Of great significance are its possible protective properties (Snodderly 1995).
Optics becomes critical however, in the operation of receptor cells, whose diameter is of the order of the wavelength of light. A start was made in the 1840s by Ernst BrĂźcke, at the time Helmholtzâs fellow student in Johannes MĂźllerâs Institute in Berlin, who made the observation that rodlike retinal receptor cells acted like light guides. He seems not to have published it; all we have is Helmholtzâs (1866) report and the comment that light once it had entered a receptor and impinged on the cylindrical boundary separating media of high from low refractive index would undergo total reflection and proceed further along the receptor and not leave it.
Receptor optics became mainstream with the discovery of the retinal directional sensitivity by Stiles and Crawford (1933) and the conjecture by Toraldo (1949) of retinal cones being wave guides. This set into motion extensive research activity, still ongoing. The state of the subject is well captured in the contributions to Enoch and Tobeyâs Vertebrate Receptor Optics (1981).
Whereas rods and their rhodopsin photopigment had been fully identified with scotopic vision, the same could not be said about cones and the cone pigments till the 1960s. Before that, because there was no firsthand knowledge of the phototransduction that underlies color vision, the possibility remained open that there was only a single cone pigment and that wavelength analysis came about through an intracellular filtering process, as indeed is the case with oil drops in birds (Walls 1942). Of historical interest, therefore, is the attempt by Ingelstam (1956) to show that the ultramicroscopic structure of receptors, which had just been discovered, might allow wavelength-dependent differential energy concentration. Most of these conjectures were laid to rest by Brindley and Rushtonâs (1959) demonstration that to the human observer colors looked the same whether light entered the retina from the front or the back. The effect of the concentration of photopigments on their absorption spectrumâcalled self-screeningâmust, however, be considered in color vision theory (Brindley 1960) and probably plays a role in the StilesâCrawford effect of the second kind (Stiles 1939), color changes associated with direction of incident light.
1.4 THE RETINAL IMAGE
1.4.1 ABERRATIONS OF THE EYE
A theoretical approach to the monochromatic aberrations in an optical system requires adequate knowledge of the optical parameters, position and curvature of the surfaces, and refractive index of the media. Because the precision needed to estimate image quality by ray tracing was lacking, this topic of visual optics was largely unattended until it was, so to speak, turned upside down quite recently by nulling out the aberrations. There was a brief flurry of activity centered on spherical aberration, when it was fingered to account for night myopia (Koomen et al. 1951), of practic...
Table of contents
- Cover
- Half Title
- Title Page
- Copyright Page
- Table of Contents
- Preface
- Editor
- Contributors
- Part I Introduction
- Part II Fundamentals
- Part III Optical properties of the eye
- Index