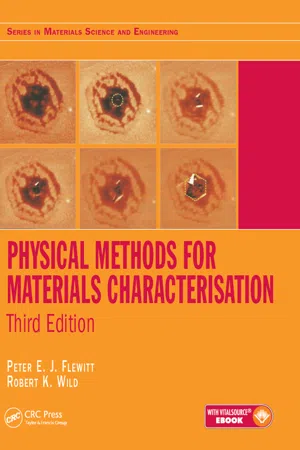
This is a test
- 725 pages
- English
- ePUB (mobile friendly)
- Available on iOS & Android
eBook - ePub
Physical Methods for Materials Characterisation
Book details
Book preview
Table of contents
Citations
About This Book
This completely revised and expanded new edition covers the full range of techniques now available for the investigation of materials structure and accurate quantitative determination of microstructural features within materials. It continues to provide the best introductory resource for understanding the interrelationship between microstructure and physical, mechanical, and chemical properties, as well as selection and application of techniques for both basic and applied studies. In particular, changes have been made to reflect developments in analysis of nanoscale and biological materials.
Frequently asked questions
At the moment all of our mobile-responsive ePub books are available to download via the app. Most of our PDFs are also available to download and we're working on making the final remaining ones downloadable now. Learn more here.
Both plans give you full access to the library and all of Perlego’s features. The only differences are the price and subscription period: With the annual plan you’ll save around 30% compared to 12 months on the monthly plan.
We are an online textbook subscription service, where you can get access to an entire online library for less than the price of a single book per month. With over 1 million books across 1000+ topics, we’ve got you covered! Learn more here.
Look out for the read-aloud symbol on your next book to see if you can listen to it. The read-aloud tool reads text aloud for you, highlighting the text as it is being read. You can pause it, speed it up and slow it down. Learn more here.
Yes, you can access Physical Methods for Materials Characterisation by Peter E. J. Flewitt, Robert K. Wild in PDF and/or ePUB format, as well as other popular books in Physical Sciences & Physics. We have over one million books available in our catalogue for you to explore.
1
INTRODUCTION
1.1 INTRODUCTION INTRODUCTION INTRODUCTION INTRODUCTION
This book provides a guide to those techniques and procedures which enable the microstructure of materials to be completely classified and characterised. As a consequence, it is appropriate to those studying and working in the interrelated fields of metallurgy, materials science, ceramics, polymer science, engineering materials, electronic engineering and solid-state physics. Material is the generic term used to describe physical matter in the solid state which occurs naturally or is manufactured to achieve particular physical properties and characteristics. Materials have been classified in various ways, but perhaps the simplest and most complete classification divides into two categories (Table 1.1) (Bever 1986), one based upon the nature of the material and the other upon the application. Such a classification is flexible, accommodating existing materials and perceived future materials. It is not appropriate to address each of the materials set out under the heading of nature in Table 1.1 in detail, but rather to consider briefly how their atomic and molecular structure influences the mechanical and physical properties associated with some of the more important of these. It is to this nanoscale level that microstructure has to be resolved ultimately, although there are essentially many lower-resolution techniques covering the mesoscale and micro-scale that assist with this understanding.
TABLE 1.1 Classification of Materials Based on Nature and Applications
Nature | Applications |
Ceramics | Industrial materials |
Glasses | Electrical materials |
Metals and alloys | Electronic materials |
Other inorganic materials (including semiconductors) | Superconducting materials |
Polymers | Magnetic materials |
Elastomers | Nuclear materials |
Fibres | Materials for other energy applications |
Composite materials | Optical materials |
Wood | Biomedical materials |
Paper and paperboard | Dental materials |
Single layer biological materials | Building materials |
Source: Bever, M.B., Encyclopedia of Materials, Science and Engineering, vol. 1, Cahn, R.W., ed., Pergamon, Oxford, UK, 1986. |
1.2 ATOM BONDING
There is an attractive force between atoms and a repulsive force which prevents them from approaching beyond a minimum distance. The stable position for the atoms is best addressed by considering how the potential energy of a pair of atoms varies with their separation. The repulsive force gives rise to a positive potential energy which results in work being done on the system to bring the atoms closer together; this energy varies as an inverse power of the atomic separation r as A/rn. The attractive force gives a negative potential energy of the form B/rm which tends to zero when the atoms are widely separated and increases negatively as they are brought together. The combined curve in potential energy, A/rn – B/rm, as a function of the interatomic spacing is shown in Figure 1.1, and this passes through a minimum. The atomic separation r0 at which this minimum potential energy occurs is the stable spacing for the pair of atoms; the negative and positive forces balance. This minimum in the potential energy arises because the power n in the repulsive term is greater than m in the attractive term. If m is less than n, an unstable situation would develop and all the atoms would collapse together.
Since the mechanical and physical properties of materials are a direct consequence of their interatomic force (Mott and Jones 1958, Cottrell 1967, 1990, Mott 1976) (Table 1.2), it is appropriate to consider the different bonding configurations that are associated with various materials and the potential crystalline and molecular structures that can form. However, it has to be remembered that the bond descriptions given here are simple and idealised. Moreover, many materials used for practical applications are in the form of either polycrystalline arrays or aggregates, rather than simple single crystals. It is the specific type of interatomic bond that leads, in solid crystals, to the development of atoms or molecules into particular periodic arrangements in three dimensions and thus specific materials. Crystals differ from liquids and gases because the atomic arrangements in the latter do not possess this periodicity. However, not all solids are crystalline; some are amorphous, such as glass, a state that does not have any long-range periodic arrangement of atoms. The regularity of the array can be described in terms of symmetry elements (Kelly and Groves 1970, Barrett and Massalski 1986), and these elements determine the directionality of the physical properties of crystals. For example, the symmetry elements reveal directions where electrical resistance in a crystal will be similar. Figure 1.2 shows a simple crystal lattice where all the cells are identical. The size and shape of the outlined unit cell can be described by three vectors, a, b and c, which define the crystallographic axes (Figure 1.3). The lengths a, b and c and the angles between them α, β and γ are the constants which describe the crystal uniquely. Figure 1.4 shows the 14 possible lattice arrangements, Bravais lattice, for crystalline materials.

FIGURE 1.1 The potential energy of two atoms as a function of their separation r. The minimum in the potential energy at a separation r0 corresponds to the equilibrium separation.
TABLE 1.2 Summary of Physical and Mechanical Properties Associated with Interatomic Bonds
Property | Ionic | Covalent | Metallic | van der Waals |
Non-directional; structures of high coordination | Directional; structures of low coordination and low density | Non-directional; structures of high coordination and high density | Analogous to metallic bond | |
Mechanical | Strong, hard crystals | Strong, hard crystals | Variable crystals | Weak, soft crystals |
Thermal | High melting point, low expansion coefficient | High melting point, low expansion coefficient | Range of melting points, extended liquidus range | Low melting point, large expansion coefficient |
Electrical | Weak insulator, conduction by ion transport when liquid | Insulator in solid and liquid states | Conduction by electron transport | Insulator |
Optical | Absorption and other properties mainly of the individual ions | High refractive index, absorption different in solid or gas | Opaque, with similar properties in a liquid state | Properties of individual molecules |
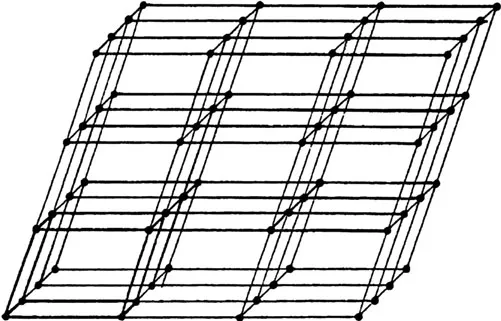
FIGURE 1.2 A simple point lattice defining a unit cell. (From Cullity, B.D.: Elements of X-ray Diffraction. 1979. Copyright Wiley-VCH Verlag GmBH & Co. KGaA. Reproduced with permission.)
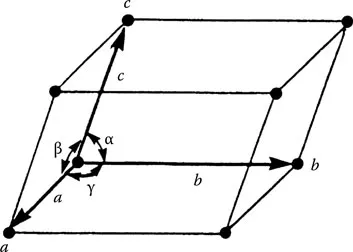
FIGURE 1.3 The unit cell can be described by three vectors a, b and c; the lattice constants...
Table of contents
- Cover
- Half Title
- Title Page
- Copyright Page
- Table of Contents
- Preface to the Third Edition
- Preface to the Second Edition
- Preface to the First Edition
- Authors
- 1 Introduction
- 2 Interaction of Radiation with Materials
- 3 Diffraction
- 4 Photo/Electromagnetic Sources
- 5 Electron Sources
- 6 Atom/Ion Sources
- 7 Data Acquisition, Processing and Interpretation
- Appendix 1: Vacuum Systems
- Appendix 2: International Standards
- Appendix 3: List of Symbols Used in Book
- Appendix 4: Reference Data
- Appendix 5: Physical Constants
- Appendix 6: Acronyms for Techniques Used in Book
- Appendix 7: Electron Structure of Elements
- Index