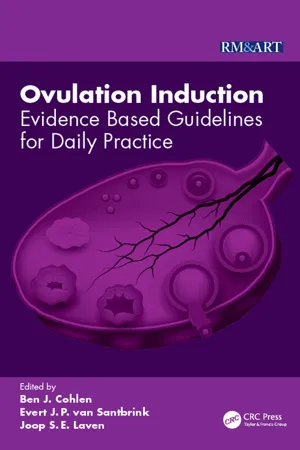
eBook - ePub
Ovulation Induction
Evidence Based Guidelines for Daily Practice
This is a test
- 192 pages
- English
- ePUB (mobile friendly)
- Available on iOS & Android
eBook - ePub
Ovulation Induction
Evidence Based Guidelines for Daily Practice
Book details
Book preview
Table of contents
Citations
About This Book
Anovulation â one of the most common causes of infertility â is here given a thorough review, with classification of the different subtypes, how they are diagnosed, how they are treated, and the possible complications and outcomes involved. This is a comprehensive evidence-based summary from an international expert team, with guidelines for daily practice clearly stated and summarized for your convenience.
Frequently asked questions
At the moment all of our mobile-responsive ePub books are available to download via the app. Most of our PDFs are also available to download and we're working on making the final remaining ones downloadable now. Learn more here.
Both plans give you full access to the library and all of Perlegoâs features. The only differences are the price and subscription period: With the annual plan youâll save around 30% compared to 12 months on the monthly plan.
We are an online textbook subscription service, where you can get access to an entire online library for less than the price of a single book per month. With over 1 million books across 1000+ topics, weâve got you covered! Learn more here.
Look out for the read-aloud symbol on your next book to see if you can listen to it. The read-aloud tool reads text aloud for you, highlighting the text as it is being read. You can pause it, speed it up and slow it down. Learn more here.
Yes, you can access Ovulation Induction by Ben J. Cohlen, Evert Van Santbrink, Joop Laven, Ben J. Cohlen, Evert Van Santbrink, Joop Laven in PDF and/or ePUB format, as well as other popular books in Medicine & Medical Theory, Practice & Reference. We have over one million books available in our catalogue for you to explore.
Information
1
Physiology of the Menstrual Cycle: Understanding the Principles of Ovarian Stimulation
Introduction
Following the pioneering days of in vitro fertilization (IVF), ovarian stimulation became an integral part of assisted reproductive techniques (ARTs). Over the last three decades, ovarian stimulation protocols have undergone numerous refinements in an effort to optimize the follicular phase of ovarian stimulation and, increasingly, the resulting luteal phase, too.
Optimal use of ovarian stimulation protocols requires a clear understanding of the physiological regulation of the ovarian cycle and the precise aims of interventions designed to mimic or override it. Moreover, as the role of the endometrium as a determinant of treatment outcomes becomes clearer, an understanding of the relationship of the cyclical phases of endometrial development is important. This chapter therefore addresses the regulation of the ovarian cycle (follicular phase, triggering of ovulation, and luteal phase) and the endometrial cycle (proliferative phase, secretory phase, and menstrual phase).
Ovarian Cycle
The Follicular Phase
The initial growth of primordial follicles (also referred to as âprimaryâ recruitment) is random, being independent of follicle-stimulating hormone (FSH). The regulation of early follicular development and atresia and the degree to which FSH influences this remains unclear (1). Studies in women with a mutated FSH beta subunit have shown follicular growth to occur up to the stage of secondary recruitment without the need for FSH. Factors such as TGF-alpha from theca cells, growth differentiation factor 9 (GDF), and bone morphogenetic protein 15 produced by the oocyte may limit the effects of FSH on granulosa cell differentiation and follicle development at this early stage (1). Throughout reproductive life, gonadotrophin-releasing hormone (GnRH) is released from the hypothalamus in a pulsatile manner and stimulates the synthesis and release of FSH and luteinizing hormone (LH) by the anterior pituitary gland.
In the non-conception cycle, due to the involution of the corpus luteum during the late luteal phase of the menstrual cycle, estradiol (E2), inhibin A, and progesterone (P) levels fall. These, in turn, cause increased slow pulse frequencies (<1 pulse per 2â3 hour) of GnRH secretion, inducing rising levels of FSH at the end of the luteal phase. Only those antral follicles that happen to be at a more advanced stage of maturation during the inter-cycle rise in FSH gain gonadotrophin dependence and continue to grow (2). This process is referred to as cyclic, gonadotropin-dependent, or âsecondaryâ recruitment. The cohort size of healthy early antral follicles recruited during the luteo-follicular transition is around 10 per ovary, based on indirect observations. The theca cells of the maturing follicle develop LH receptors and synthesize androstenedione while the granulosa cells respond to FSH and synthesize aromatase enzyme, which, in turn, converts the androstenedione to estradiol (E2) (the two cellâtwo gonadotrophin theory) (3,4).
Inhibin B, secreted by the recently recruited cohort of follicles in response to FSH, rapidly rises immediately after the inter-cycle rise in FSH (2). This rise in inhibin B, along with the rising estradiol (E2) levels during the mid and late follicular phase, has negative feedback at the hypothalamicâpituitary axis causing a steady decrease in serum FSH levels. This secures the selection of a single dominant follicle, which becomes less dependent on FSH and continues to grow in a natural cycle. The remaining follicles from the recruited cohort cease to mature and undergo atresia through a process of apoptosis (Figures 1.1 and 1.2).
Decreasing follicular phase FSH levels (effectively restricting the time when FSH levels remain above the threshold, referred to as the FSH window) is crucial for selecting a single dominant follicle from the recruited cohort. The key role of FSH in stimulating preovulatory follicle growth has been demonstrated by the use of exogenous FSH to stimulate follicle growth up to the preovulatory stage in hypophysectomized women (1,4). Although granulosa cells from early antral follicles respond only to FSH, those from mature follicles (exhibiting receptors to both gonadotropins) are responsive to both FSH and LH. The maturing dominant follicle may become less dependent on FSH because of the ability to respond to LH (1).
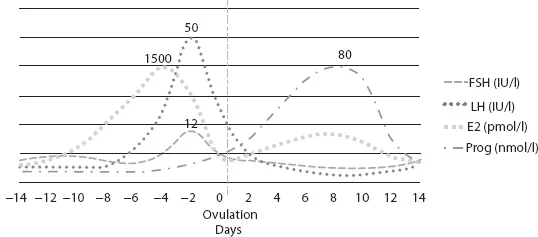
FIGURE 1.1 Graphic representation of variation in pituitary and ovarian hormonal levels during menstrual cycle (not to scale).
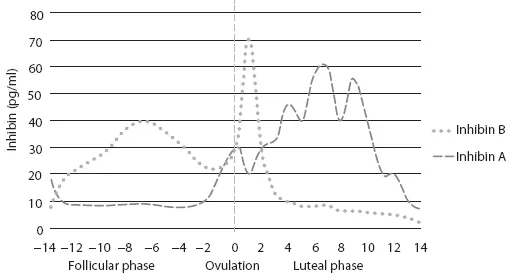
FIGURE 1.2 Graphic representation of inhibin A and B levels during menstrual cycle (not to scale) (2,3).
The LH Surge and the Luteal Phase
Rising levels of E2, produced by the granulosa cells of the preovulatory dominant follicle, cause the release of fast pulse frequencies (>1 pulse per hour) and high amplitude GnRH, favoring increased LH production (2). This causes a switch from a negative to positive feedback effect, resulting in a rapid rise in LH release and the so-called LH surge. The initial onset of the LH surge (not the peak level, which is reached 10â12 hours before ovulation) induces the ovulation about 34â36 hours later. The mean duration of the LH surge is 48 hours. Besides triggering ovulation, the LH surge induces the formation of the corpus luteum, for which an adequate amplitude and duration of LH surge is essential. The wall of the follicle collapses, and capillaries invade the developing corpus luteum probably under the influence of angiogenic and mitogenic factors. The differentiated granulosa cells in the corpus luteum will produce progesterone (P) in increasing amounts, E2, and inhibin A (1,2).
In some species, such as rodents, prolactin-like hormones play a principal role in the luteotropic process, and luteal regression involves a uterine signal such as prostaglandin F2 alpha (1). In contrast to this, in humans, LH is the principle hormone responsible for the following events. First, the mid-cycle surge of gonadotropins (notably, LH) stimulates the resumption of oocyte meiotic maturation; rupture of the dominant follicle, allowing the release of oocyte (ovulation), and corpus luteum formation (i.e., luteinization). Second, the pulsatile secretion of pituitary LH during the luteal phase of the menstrual cycle promotes the continued development and normal functional lifespan of the corpus luteum (1,2).
And finally, the exponential rise in circulating levels of the LH-like hormone, chorionic gonadotropin (CG), secreted by the implanting blastocyst and syncytiotrophoblast of the developing placenta, extends the functional lifespan of the corpus luteum in early pregnancy until luteal activities are assumed by the placenta, that is, at the lutealâplacental shift (1,4).
Studies during the 1970s and 1980s and more recent experiments using GnRH antagonists and pure recombinant human LH or human CG (hCG) have strengthened the critical role of LH/CG in regulating primate luteal structureâfunction and is increasingly being applied in ovarian stimulation for IVF (1).
Although the maturing dominant follicle may be less sensitive to acute LH withdrawal at mid-cycle, a GnRH-induced LH surge of substantial length is required for ovulation and development of normal luteal function. What remains unclear is how duration and/or amplitude of the mid-cycle LH surge influences peri-ovulatory events. Initial monkey and human studies on GnRH-induced LH surges or administering exogenous LH/CG suggest that surges of lesser duration (<24 hours) and amplitude are sufficient to reinitiate oocyte meiosis and granulosa cell luteinization, but surges of greater duration (>24 hours) and amplitude improve oocyte recovery, fertilization, and corpus luteum development (1,2). Although the LH surge is believed to be the physiological signal for peri-ovulatory events, studies in rodents showed that a mid-cycle bolus of FSH can replace LH and elicit oocyte maturation, ovulation, and successful pregnancy (1). The clinical relevance of these physiological observations has recently been brought into sharper focus by the use of GnRHa agonists to induce a mid-cycle LH surge in IVF cycles. Although the short period of stimulation reduces the risk of developing ovarian hyperstimulation syndrome, it is clear that it may impact detrimentally on the luteal phase, compared with the use of hCG as a trigger, which remains bioactive for a longer period. These clinical issues are addressed elsewhere.
The LH-stimulated luteinization of granulosa cells around ovulation includes enhanced vascular endothelial growth factor (VEGF) production, which is likely essential for the angiogenic process within the corpus luteum. With regards to hCG, a mid-cycle bolus in ovarian stimulation cycles increased expression of the endogenous angiopoietin agonist, Ang-1, without altering that of the endogenous antagonist, Ang-2, in macaque granulosa cells. These factors control not only the development or maintenance of the vasculature in developing tissue beds, but also vascular integrity, maturity, and permeability (1). It has been proposed that overexpression, increased bioavailability, or a change in the ratio of angiogenic factors, notably VEGF-A, is a cause of ovarian hyperstimulation syndrome (OHSS), a serious side effect of ovarian stimulation characterized by intravascular volume loss and extravascular fluid accumulation. The early or late occurrence of OHSS in ovarian stimulation cycles has been linked to the ovulatory hCG bolus and endogenous CG production at pregnancy recognition, respectively (1,2).
The Endometrium
The basalis layer that remains after shedding of the more superficial endometrium at menstruation needs to regrow and differentiate (proliferative phase) under the E2 influence secreted by the growing follicles in the follicular phase of the ovarian cycle. The highest E2 response is in the glands via increased E2 receptor (ER) expression (4). First, there is an increase in the mitotic activity, and second, there is formation of a loose capillary network in the spiral vessels. After ovulation, the progesterone (P) produced by the corpus luteum causes the cessation of endometrial proliferation and initiates glandular secretion (secretory phase). The endometrial glands become tortuous and spiral vessels coiled. During the secretory phase, a short specific period of uterine receptivity toward embryonic implantation is designated as the âimplantation windowâ (2...
Table of contents
- Cover
- Half Title
- Title Page
- Copyright Page
- Table of Contents
- Foreword
- Evidence Evaluation
- Contributors
- 1. Physiology of the Menstrual Cycle: Understanding the Principles of Ovarian Stimulation
- 2. Classification of Anovulation
- 3. Causes of Anovulation: WHO Class 1
- 4. Causes of Anovulation: Normogonadotropic Normoestrogenic Anovulation Non-PCOS
- 5. Causes of Anovulation: WHO Type 2: Polycystic Ovary Syndrome
- 6. Causes of Anovulation: WHO Class 3
- 7. Other Endocrine Disorders Causing Anovulation: Congenital Adrenal Hyperplasia
- 8. Other Endocrine Disorders Causing Anovulation: Thyroid Disorders
- 9. Other Endocrine Disorders Causing Anovulation: Prolactinomas
- 10. Genetics of Anovulation
- 11. Diagnosis of Anovulation
- 12. Ovulation Induction versus Controlled Ovarian Hyperstimulation
- 13. Monitoring of Ovulation Induction Cycles
- 14. Treatment of WHO 1: GnRH or Gonadotropins?
- 15. Treatment of WHO 2: Clomiphene Citrate
- 16. Treatment of WHO 2: Aromatase Inhibitors
- 17. Treatment of WHO 2: Insulin Sensitizers
- 18. Treatment of WHO 2: Laparoscopic Electrocautery of the Ovaries
- 19. Treatment of WHO 2: Gonadotropins and the Role of GnRH Agonists and Antagonists
- 20. Treatment of WHO 2: Lifestyle Modifications
- 21. Treatment of WHO 3
- 22. How to Define Success in Ovulation Induction
- 23. Complications of Ovarian Stimulation: Multifollicular Development
- 24. Complications of Ovulation Induction: Ovarian Hyperstimulation Syndrome
- 25. Complications of Ovarian Stimulation: Multiple Pregnancies
- 26. Long-Term Complications of Anovulation
- 27. Predicting Outcome of Treating Anovulation
- 28. Future Prospects
- 29. Summary: Levels of Evidence of Statements
- 30. Summary: Grade of Strength of Recommendations
- Index