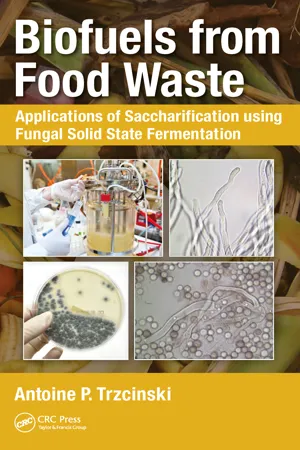
eBook - ePub
Biofuels from Food Waste
Applications of Saccharification using Fungal Solid State Fermentation
This is a test
- 125 pages
- English
- ePUB (mobile friendly)
- Available on iOS & Android
eBook - ePub
Biofuels from Food Waste
Applications of Saccharification using Fungal Solid State Fermentation
Book details
Book preview
Table of contents
Citations
About This Book
According to the UN's Food & Agricultural Organization (FAO), one third of food produced globally for human consumption (nearly 1.3 billion tons) is lost annually. Food waste has often been incinerated with other combustible municipal wastes for possible recovery of heat or other forms of energy, however, incineration is not cost-effective, and can cause air pollution. Due to its organics- and nutrient-rich nature, food waste could be viewed as a useful resource for production of high-value platform chemicals through fermentation. This book examines the bioconversion of food wastes to energy and the recent developments in ethanol, hydrogen, methane, and biodiesel production from food wastes.
Frequently asked questions
At the moment all of our mobile-responsive ePub books are available to download via the app. Most of our PDFs are also available to download and we're working on making the final remaining ones downloadable now. Learn more here.
Both plans give you full access to the library and all of Perlegoâs features. The only differences are the price and subscription period: With the annual plan youâll save around 30% compared to 12 months on the monthly plan.
We are an online textbook subscription service, where you can get access to an entire online library for less than the price of a single book per month. With over 1 million books across 1000+ topics, weâve got you covered! Learn more here.
Look out for the read-aloud symbol on your next book to see if you can listen to it. The read-aloud tool reads text aloud for you, highlighting the text as it is being read. You can pause it, speed it up and slow it down. Learn more here.
Yes, you can access Biofuels from Food Waste by Antoine Prandota Trzcinski in PDF and/or ePUB format, as well as other popular books in Physical Sciences & Industrial & Technical Chemistry. We have over one million books available in our catalogue for you to explore.
Information
1
Bioconversion of Food Wastes to Energy
1.1Introduction
Food waste (FW) is organic waste discharged from various sources including food processing plants, and domestic and commercial kitchens, cafeterias, and restaurants. According to FAO (2012), nearly 1.3 billion tonnes of foods including fresh vegetables, fruits, meat, and bakery and dairy products are lost along the food supply chain. The amount of FW has been projected to increase in the next 25 years due to economic and population growth, mainly in Asian countries. For example, the annual amount of urban FW in Asian countries could rise from 278 to 416 million tonnes from 2005 to 2025 (Melikoglu et al., 2013b). Typical foods wasted in AsiaâPacific countries and around the world are summarized in Table 1.1 (FAO, 2012).
Table 1.1
Typical Wasted Foods in Several AsiaâPacific Countries and around the Globe


FW is traditionally incinerated with other combustible municipal wastes for generation of heat or energy. It should be recognized that FW contains high levels of moisture, which may lead to the production of dioxins during its combustion together with other wastes of low humidity and high calorific value (Katami et al., 2004). In addition, incineration of FW can potentially cause air pollution and loss of chemical values of FW. These suggest that an appropriate management of FW is strongly needed (Ma et al., 2009a). FW is mainly composed of carbohydrate polymers (starch, cellulose, and hemicelluloses), lignin, proteins, lipids, organic acids, and a remaining, smaller inorganic part (Table 1.2). Hydrolysis of carbohydrate in FW may result in the breakage of glycoside bonds with releasing polysaccharides as oligosaccharides and monosaccharides, which are more amenable to fermentation. Total sugar and protein contents in FW are in the range of 35.5%â69% and 3.9%â21.9%, respectively. As such, FW has been used as the sole microbial feedstock for the development of various kinds of value-added bioproducts, including methane, hydrogen, ethanol, enzymes, organic acid, biopolymers, and bioplastics (Han and Shin, 2004; Rao and Singh, 2004; Wang et al., 2005a; Sakai et al., 2006; Yang et al., 2006; Ohkouchi and Inoue, 2007; Pan et al., 2008; Koike et al., 2009; He et al., 2012b; Zhang et al., 2013b). Fuel applications ($200â400/ton biomass) usually create more value compared to generating electricity ($60â150/ton biomass) and animal feed ($70â200/ton biomass). Due to inherent chemical complexity, FW also can be utilized for production of high-value materials, such as organic acids, biodegradable plastics, and enzymes ($1000/ton biomass) (Sanders et al., 2007). However, it should be noted that the market demand for such chemicals is much smaller than that for biofuels (Tuck et al., 2012). Therefore, this chapter intends to review the FW valorization techniques that have been developed for the production of various biofuels, such as ethanol, hydrogen, methane, and biodiesel.
Table 1.2
Composition of Mixed Food Waste

1.2Ethanol Production
Recently, global demand for ethanol has increased due to its wide industrial applications. Ethanol is mainly used as a chemical feedstock to produce ethylene with a market demand of more than 140 million tonnes per year, a key material for further production of polyethylene and other plastics. As such, bioethanol produced from cheap feedstocks has gained interest (Lundgren and Hjertberg, 2010; International Renewable Energy Agency, 2013). Traditionally, bioethanol is produced from cellulose and starch rich crops, for example, potato, rice, and sugar cane (Thomsen et al., 2003). Starch can be easily converted to glucose by commercial enzymes and subsequently fermented to ethanol particularly by Saccharomyces cerevisiae. However, the hydrolysis of cellulose is more difficult. FW hydrolysis becomes much harder if large quantities of cellulosic feedstocks are present in FW. Use of abundant and cheap wastes such as lignocellulosic, municipal, and FW has been explored as alternative substrates for ethanol production (Kim and Dale, 2004; Jensen et al., 2011).
1.2.1Pretreatments
Harsh pretreatment may not be necessary during the conversion of FW to ethanol prior to enzymatic hydrolysis (Kumar et al., 1998; Tang et al., 2008). Instead, autoclave of FW before fermentation is often required for improving product yield and purity, but at the cost of energy and water consumption. It should be noted that thermal treatment may lead to partial degradation of sugars and other nutritional components, as well as side reactions (e.g., Maillard reactions) through which the amounts of useful sugars and amino acids are reduced (Sakai and Ezaki, 2006). Moreover, fresh and wet FW appear to be more effective than rewetted dried FW (Kim et al., 2005). This is mainly due to the decreased specific surface area of the dried substrate, resulting in a decrease in the reaction efficiency between the enzymes and substrate. Therefore, the utilization of FW without a drying pretreatment is preferred as long as microbial contamination is manageable. Without thermal sterilization, acidic condition is needed to prevent microbial contamination and putrefaction (Ye et al., 2008a; Koike et al., 2009). As such, acid-tolerant ethanol-producing microorganisms such as Zymomonas mobilis have been employed for the fermentation of FW (Tao et al., 2005; Wang et al., 2008a).
1.2.2Saccharification
The conversion efficiency of FW to ethanol depends on the extent of carbohydrate saccharification as yeast cells cannot ferment starch or cellulose directly into bioethanol (Tubb, 1986). A mixture of ι-amylase, β-amylase, and glucoamylase of various origins is more effective for substrates with higher molecular weight. Pullulanase has also been added to the list of saccharifying enzymes recently (Tomasik and Horton, 2012). As a direct endo-acting debranching enzyme, pullulanase can specifically catalyze the hydrolysis of ι-1,6-glucosidic linkages of branched polysaccharides (e.g., pullulan, dextrin, amylopectin, and related polymers), resulting in the release of linear oligosaccharides. Small fermentable sugars (e.g., maltose, amylose, glucose, maltose syrups, and fructose) can be produced in saccharification process, whereas cellulases and xylanases including endoglucanase, exoglucanase, β-glucosidase, and β-xylosidase, can also be employed to improve the hydrolysis of cereals for conversion of starches to glucose (Ducroo, 1987).
Table 1.3 shows the glucose and ethanol yields of different types of FW. The highest glucose concentration of about 65 g reducing sugar (RS)/100 g FW was obtained with ι-amylase at a dose of 120 U/g dry substrate, glucoamylase (120 U/g dry substrate), cellulase (8 FPU/g dry substrate), and β-glucosidase (50 U/g dry substrate) (Cekmecelioglu and Uncu, 2013). In a study by Hong and Yoon (2011), a mixture of commercial enzymes consisting of ι-amylase, glucoamylase, and protease resulted in 60 g RS/100 g FW.
Table 1.3
Ethanol Production from Food Wastes


1.2.3Process Configurations
High glucose yield is achievable by increasing enzyme concentration and temperature at different solid loads, agitation speeds, and hydrolysis times in the saccharification processes (Sharma et al., 2007; Ado et al., 2009; Shen et al., 2009; Zhang et al., 2010). High glucose concentration may result in catabolite repression of the enzymes (Oberoi et al., 2011b). Therefore, fed-batch and simultaneous saccharification and fermentation methods have been developed ...
Table of contents
- Cover
- Half Title
- Title Page
- Copyright Page
- Contents
- Author
- Chapter 1: Bioconversion of Food Wastes to Energy
- Chapter 2: Platform Chemical Production from Food Wastes
- Chapter 3: Enzyme Production from Food Wastes
- Chapter 4: Enhanced Glucoamylase Production by Aspergillus awamori Using Solid State Fermentation
- Chapter 5: Enhancing the Hydrolysis and Methane Production Potential of Mixed Food Wastes by an Effective Enzymatic Pretreatment
- References
- Index