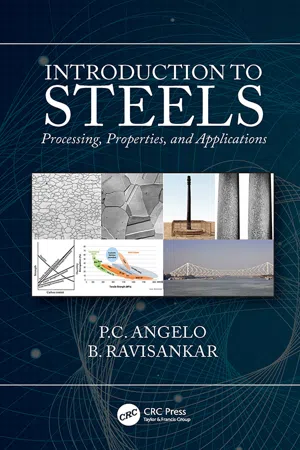
eBook - ePub
Introduction to Steels
Processing, Properties, and Applications
This is a test
- 154 pages
- English
- ePUB (mobile friendly)
- Available on iOS & Android
eBook - ePub
Book details
Book preview
Table of contents
Citations
About This Book
The book briefly describes the structure, properties and applications of various grades of steel, primarily aimed at non-metallurgical students from other engineering streams. The book consists of nine chapters covering most of the important types of steels and their physical metallurgy, microstructure and engineering applications including iron-carbon diagram, heat treatment, surface hardening methods, effect of alloying, specific applications, selection of materials, case studies and so forth. The book also contains subjective and objective questions aimed at exam preparation.
Key Features
-
- Exclusive title aimed at introduction to steels for non-metallurgy audience
-
- Includes microstructure, composition, and properties of all the most commonly used steels
-
- Describes the heat treatments and the required alloying additions to process steel for the intended applications
-
- Discusses effects of alloying elements on steel
-
- Explores development of steels for specialized areas such as the automobile, aerospace, and nuclear industries
Frequently asked questions
At the moment all of our mobile-responsive ePub books are available to download via the app. Most of our PDFs are also available to download and we're working on making the final remaining ones downloadable now. Learn more here.
Both plans give you full access to the library and all of Perlego’s features. The only differences are the price and subscription period: With the annual plan you’ll save around 30% compared to 12 months on the monthly plan.
We are an online textbook subscription service, where you can get access to an entire online library for less than the price of a single book per month. With over 1 million books across 1000+ topics, we’ve got you covered! Learn more here.
Look out for the read-aloud symbol on your next book to see if you can listen to it. The read-aloud tool reads text aloud for you, highlighting the text as it is being read. You can pause it, speed it up and slow it down. Learn more here.
Yes, you can access Introduction to Steels by P.C. Angelo, B. Ravisankar in PDF and/or ePUB format, as well as other popular books in Technologie et ingénierie & Ingénierie minière. We have over one million books available in our catalogue for you to explore.
Information
1
Iron-Carbon Diagram

1.0 Introduction
Steel is frequently the “gold-standard” even among the emerging structural materials. Steels have numerous uses. Steel is used more than any other metal for producing alloys. In 1778 the first iron bridge was built. In 1788, iron water pipe lines were laid. In 1818 the first steel ship was launched. In 1889 Gustav Eiffel, a French engineer, built the Eiffel Tower with steel. Eiffel’s contemporaries thought that his 300-meter latticed structure would prove too fragile to last. But Eiffel argued that his creation would stand at least for a quarter of a century. Even today the Eiffel Tower – the true landmark of Paris – is intact and attracting visitors from all over the world.
Steel is a moving standard, since regular and exciting discoveries are being made. This makes steel to remain the most successful and cost-effective of all materials ever. Major reason for the overwhelming dominance of steel is the variety of microstructures and properties that can be generated by solid-state transformation and processing. Therefore, in studying advanced steels, it is useful to discuss, first the nature and behavior of pure iron, then the iron-carbon alloys, and finally the complexities that arise when further solutes are added.
At least four allotropes of iron occur naturally in bulk form: body-centred cubic (bcc, α and δ, ferrite), face-centred cubic (fcc, γ, austenite) and hexagonal close packed (hcp, ε). As molten iron cools past its freezing point of 1538°C, it crystallizes into a body-centered cubic (bcc) δ allotrope. As it cools further to 1394°C, it changes into a face-centered cubic (fcc) γ-iron allotrope known as austenite. At 912°C and below, the crystal structure again becomes bcc α-iron allotrope, or ferrite. At pressures above approximately 10 GPa and temperatures of a few hundred Kelvin or less, α-iron changes into a hexagonal close-packed (hcp) structure that is also known as ε-iron; the higher-temperature γ-phase also changes into ε-iron, but does so at still higher pressure. The phase diagram for pure iron is illustrated in Figure 1.1.
The phase β in the alphabetical sequence α, β, γ, δ … is missing because the magnetic transition in ferrite was at one time incorrectly thought to be the β allotrope of iron. In fact, there are magnetic transitions in all allotropes of iron.
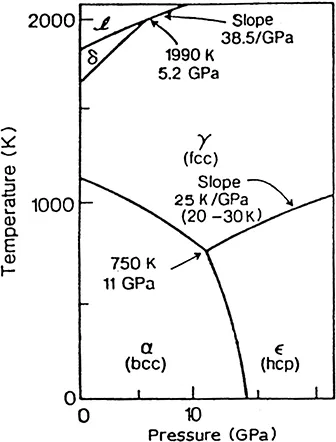
FIGURE 1.1
The phase diagram for pure iron
Some controversial experimental evidence exists for a fifth natural allotrope of iron in the core of the earth, where the pressure reaches some three million times that at the surface and where the temperature is estimated to be about 6000°C. The core of the earth is predominantly iron, and consists of a solid inner core surrounded by a liquid outer core. Knowledge of the core is uncertain, but it has been suggested that the crystal structure of the solid core may be an orthorhombic or double hcp, also denoted as β (not to be confused with β named for magnetic transition for ferrite). Calculations that assume pure iron, indicate that the ε iron remains the most stable under inner-core conditions. These high-pressure phases of iron are of no practical importance, but are important as the end member models for the solid parts of planetary cores.
Pure iron (99.99%) is not an easy material to produce and also, iron of high purity is extremely weak; the resolved shear stress of a single crystal of iron at room temperature is as low as 10 MPa, while the yield stress of a polycrystalline sample of iron at the same temperature is well below 50 MPa and has a hardness of 20–30 Brinell. But pure iron has nevertheless been made with a total impurity content of less than 60 parts per million (ppm), of which 10 ppm is accounted for by non-metallic impurities such as carbon, oxygen, sulfur and phosphorus, with the remainder representing metallic impurities. With the controlled amount of nonmetallic impurities especially carbon (between 0.002% and 2.1%) produces steel that may be up to 1000 times harder than pure iron. Maximum hardness of 65 HRc is achieved with 0.6% carbon content. The possibility of achieving a variety of microstructure due to the allotropic behavior of iron, good workability (deformability) due to the softness of iron and the strength achieved due to carbon, make the steel suitable for a wide variety of applications. Steel is an enigma as it rusts easily, yet it is the most important of all metals. Steel is 90% of all metals being refined today as steel is useful in terms of its mechanical, physical and chemical properties.
It is well known that steel is an interstitial solid solution (alloy) of iron and carbon and it is more often referred to as a metal and while with subsequent addition of other solutes it is called as alloy steel, though it is a misnomer. Alloy steels are by far the most common industrial metals as they have a great range of desirable properties. Steel, with smaller carbon content than pig iron (about 4 wt.% C) but more than wrought iron (almost a pure iron), was first produced in antiquity by using a bloomery. By 1000 BCE, blacksmiths in Luristan in western Persia were making good steel. The improved versions such as Wootz steel by India and Damascus steel were developed around 300 BCE. These methods were specialized, and so steel did not become a major commodity until 1850s. New methods of producing it by carburizing bars of iron in the cementation process were devised in the 17th century. In the Industrial Revolution, new methods of producing bar iron without charcoal were devised and these were later applied to produce steel. In the late 1850s, Henry Bessemer invented a new steelmaking process, involving blowing air through molten pig iron, to produce mild steel. This made steel much more economical, thereby leading to wrought iron being no longer produced in large quantities. Carbon steels are least expensive of all metals while stainless steels are costly.
1.1 Iron-Carbon Diagram
Of all the binary alloy systems, the one that is possibly the most important is iron and carbon. Both steels and cast irons, primary structural materials in every technologically advanced culture, are essentially iron–carbon alloys. This section deals with the study of the phase diagram and the solidification and development of several of the possible microstructures in iron-carbon alloys.
Iron–carbon phase diagram is presented in Figure 1.2. Pure iron, upon heating, experiences two changes in crystal structure before it melts. At room temperature the stable form, called alpha ferrite, or iron, has a BCC crystal structure. Ferrite experiences a polymorphic transformation to FCC austenite, or iron, at 912°C. This austenite persists up to 1394°C; at that temperature the FCC austenite reverts back to a BCC phase known as delta ferrite that finally melts at 1538°C. All these changes are shown along the left vertical axis of the phase diagram.
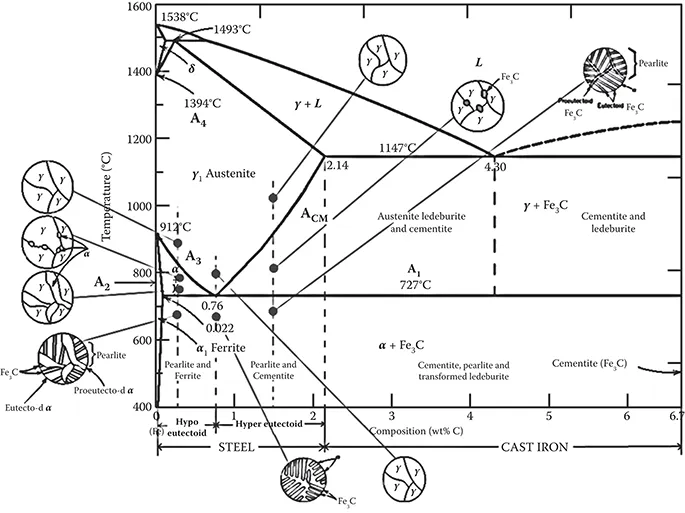
FIGURE 1.2
Iron-carbon (iron-iron carbide) phase diagram
The composition axis in Figure 1.2 extends only to 6.70 % C as at this concentration the intermediate compound iron carbide, or cementite (Fe3C), is formed that is represented by a vertical line on the phase diagram. Thus, the iron-carbon system may be divided into two parts: an iron-rich portion, as in Figure 1.2; and the other (not shown) for compositions between 6.70 and 100% C (pure graphite). In practice, all steels and cast irons have carbon contents less than 6.70% C and therefore, only the iron–iron carbide system is considered. Figure 1.2 should be more appropriately labeled as Fe–Fe3C phase diagram, as Fe3C is now considered to be a component. Convention and convenience dictate that composition be expressed in “wt% C” rather than “wt% Fe3C”; 6.70 wt% C corresponds to 100 wt% Fe3C. In this book, all percentages refer only to wt% unless otherwise mentioned.
Carbon is an interstitial impurity in iron and forms a solid solution with ferrites, and also with austenite, as indicated in Figure 1.2. In the BCC ferrite, only small concentrations of carbon are soluble; the maximum solubility is 0.022% at 727°C. The limited solubility is explained by the shape and size of the BCC interstitial positions that make it difficult to accommodate the carbon atoms. Even though present in relatively low concentrations, carbon significantly influences the mechanical properties of ferrite. This particular iron–carbon phase is relatively soft, may be made magnetic at temperatures below 768°C. Figure 1.3 (a) is a photomicrograph of ferrite.
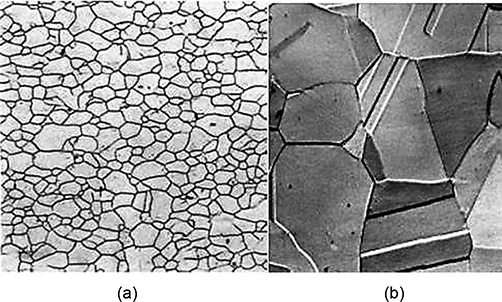
FIGURE 1.3
Photomicrograph of (a) ferrite and (b) austenite
The austenite, or gamma phase of iron, which is nonmagnetic, when alloyed with just carbon, is not stable below 727°C, as indicated in Figure 1.2. The maximum solubility of 2.14% carbon in austenite, occurs at 1147°C. This solubility is approximately 100 times greater than the maximum for BCC ferrite, as the FCC interstitial positions are larger, and therefore, the strains imposed on the surrounding iron atoms are much lower. Also the phase transformations involving austenite are very important in the heat treating of steels. Figure 1.3 (b) shows a photomicrograph of austenite phase.
The delta ferrite is virtually the same as alpha ferrite, except for the range of temperatures over which each exists. Because the delta ferrite is stable only at relatively high temperatures, it is of no technological importance and hence is not discussed further.
Cementite (Fe3C) forms when the solubility limit of carbon in ferrite is exceeded below 727°C (for compositions within the Fe3C phase region). As indicated in Figure 1.2, Fe3C will also coexist with the phase between 727 and 1147°C. Cementite is very hard and brittle; the strength of some steels is greatly enhanced by its presence. Strictly speaking, cementite is only metastable; that is, it will remain as a compound indefinitely at room temperature. But if heated to between 650 and 700°C for several years, it will gradually change or transform into iron and carbon in the form of graphite that will remain upon subsequent cooling to room temperature. Thus, the phase diagram in Figure 1.2 is not a true equilibrium one because cementite is not an equilibrium compound. However, in as much as the decomposition rate of cementite is extremely sluggish, virtually all the carbon in steel will be as Fe3C instead of graphite, and the iron–iron carbide phase diagram is, for all practical purposes, valid.
According to the iron-carbon diagram, alloys with less than 2% carbon are known as steels and alloys with more than 2% carbon are cast irons. There are three invariant (phase) reactions occurring in the iron-carbon system:
(i) A peritectic reaction at 1493°C at 0.16% carbon
delta ferrite + Liquid ↔ austenite(0.08%C) (0.5%C) (0.18%C)
This reaction is of minor importance in steels although it has some importance in welding of austenitic stainless steels, which will be discussed later in this book.
(ii) The second one is the eut...
Table of contents
- Cover
- Half Title
- Title Page
- Copyright Page
- Table of Contents
- Preface
- Author Bios
- 1. Iron-Carbon Diagram
- 2. Heat Treatment of Steels
- 3. Surface Hardening
- 4. Plain Carbon Steels
- 5. Effect of Alloying Elements in Steel
- 6. Low Alloy Steels
- 7. High Strength Steels
- 8. High Alloy Steels
- 9. Selection of Materials
- Subjective Questions
- Bibliography
- Index