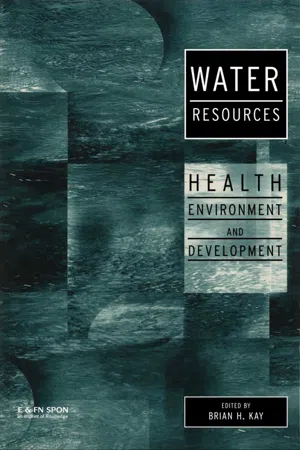
This is a test
- 272 pages
- English
- ePUB (mobile friendly)
- Available on iOS & Android
eBook - ePub
Book details
Book preview
Table of contents
Citations
About This Book
This collection addresses the complexities of water management and the impact of environmental developments such as dams, reservoirs and irrigation schemes on public health.The main focus of the book is on vector-borne diseases such as malaria, arboviruses (dengue and encephalitides) and snail- borne schistosomiasis. These are examined from a wide
Frequently asked questions
At the moment all of our mobile-responsive ePub books are available to download via the app. Most of our PDFs are also available to download and we're working on making the final remaining ones downloadable now. Learn more here.
Both plans give you full access to the library and all of Perlegoâs features. The only differences are the price and subscription period: With the annual plan youâll save around 30% compared to 12 months on the monthly plan.
We are an online textbook subscription service, where you can get access to an entire online library for less than the price of a single book per month. With over 1 million books across 1000+ topics, weâve got you covered! Learn more here.
Look out for the read-aloud symbol on your next book to see if you can listen to it. The read-aloud tool reads text aloud for you, highlighting the text as it is being read. You can pause it, speed it up and slow it down. Learn more here.
Yes, you can access Water Resources by Brian Kay in PDF and/or ePUB format, as well as other popular books in Biological Sciences & Environmental Science. We have over one million books available in our catalogue for you to explore.
Information
Chapter 1
Reservoirs: environmental process, management and policy
Walter Rast and Jeffrey A. Thornton
1.1 Introduction
Reservoirs, or artificial lakes, are a group of waterbodies that share many of the same characteristics as natural lakes yet differ from them in several important ways, not the least of which include aspects of their environmental processes and their potential to be managed. Reservoirs are located in both water-poor and water-rich regions. In the former, reservoirs are a practical way of storing surface water during times of plenty for later use during times of scarcity. In the latter, reservoirs act as storage areas to slow the passage of water downstream, reduce flood heights, and protect people and property. Many reservoirs fulfil multiple roles, such as hydropower generation, water supply for industrial, agricultural and domestic consumption, and fisheries production, and many are located in relatively close proximity to human settlements. In every case, reservoirs fulfil a critical human need for fresh water, and, in so doing, ensure their continued place in the spectrum of water resources.
1.2 Types and uses of reservoirs
The term reservoir includes many different types of constructed lakes and storage facilities. In this chapter, the term is used to describe bodies of water created by the construction of a dam or other structure specifically designed to create a pool or reservoir of surface water for any number of human purposes. These waterbodies include river-run lakes, created by constructing a barrier perpendicular to the flow of a river, and off-river storages, created by building an enclosure parallel to a stream and supplied with water by either gravity flow or pumping from the river. Figure 1.1 illustrates these basic reservoir types in a schematic way. Variants of these basic forms of reservoirs include cascades, which consist of a series of reservoirs along a single watercourse, and interbasin transfer schemes, which are designed to move water through a series of reservoirs, tunnels and canals from one watershed to another. These latter systems become especially significant in supplying water to major population centres in waterpoor areas, such as south-eastern Australia, south-western USA, north-eastern South Africa, and northern Namibia.
The principal functions of reservoirs have historically been management of water quantity, such as in water supply reservoirs and flood control reservoirs, and power generation. Reservoirs have been used in various forms to train (and drain) watercourses and riverine floodplains to permit agricultural development and promote public safety since the earliest days of human civilization. In this regard, William Dugdale, the eighteenth century English author of History of Imbanking and Drainage, cites the construction of barriers along the River Nile by the ancient Egyptians as the earliest example of such artificial water control structures. Later, the Romans built elaborate water diversion schemes to supply water to their cities, some of which (e.g. the Proserpina Dam and Cornalbo Reservoir near Merida, Spain, built in the second century) still exist. Perhaps the first modern use of dams to store surface water dates from thirteenth century Europe where weirs were constructed to provide an adequate hydraulic head, or elevation, and supply of water to drive waterwheels and supply power to mills, which were often located adjacent to streams for this reason. Subsequently, permanent settlements were often established around these mills, many of which are now long forgotten, although the cities remain. Between the fourteenth and sixteenth centuries, the uses of these constructs expanded to include facilitation of river travel by incorporating dams into canal and river navigation schemes, and flood control schemes, and spawned the first multiple use schemes with the development of artisanal fisheries within the basins created by these control structures. The first pollution problems associated with urban reservoirs were reported in the River Thames in the early seventeenth century.
By the middle of the nineteenth century, reservoirs were a common feature of the European (and North American) landscape, and since then dams (and their associated reservoirs) have featured increasingly in economic development schemes throughout the world. Many of the largest dams were built in developing countries after the Second World War. For example, the ten largest reservoirs, both in terms of volume of water impounded and height of wall, were all built subsequent to 1955, primarily in developing countries and in countries with economies in transition. Twenty-three countries had more than 100 reservoirs in active operation in 1982, with more than half of the total being located in China, which, in contrast to most other countries in these closing years of the twentieth century, is continuing to pursue a vigorous programme of new construction.

Figure 1.1 Types of reservoirs, illustrating some of their distinguishing characteristics, in a hypothetical watershed.
1.3 Characteristics of reservoirs
The characteristics of reservoirs are best described in contrast to natural lakes. One of the first observations that can be made in this regard is that reservoirs are of much more recent origin than natural lakes. As noted above, modern reservoirs date only from about the second century. Their lifespan can be documented in historical time rather than in the geological time-scale applied to most natural lakes. This, together with the fact that they have been created in areas where no natural lakes (or, at least, no significant natural lakes) previously existed, has profound implications for the environment. The exceptions to this general statement are the principally European and North American reservoirs that were created to increase the hydraulic head of natural lakes for the purposes of hydropower generation or river navigation. The consequences of such construction range from social impacts associated with the relocation of people to climatic impacts associated with the enhanced presence of water.
In contrast to natural lakes, reservoirs are often located at the terminal end of their watersheds rather than in the central part of their drainage basins. In addition, the drainage areas of reservoirs often tend to be significantly larger than the drainage areas of natural lakes. This feature ensures that reservoirs are generally subjected to greater contaminant loads than natural lakes. Although the mass of a given pollutant per unit area of watershed is usually similar in both cases, the greater watershed areas of the reservoirs will contribute a greater mass of the pollutant to the waterbodies. This feature not only explains the greater degree of enrichment experienced in many reservoirs, but also contributes to the apparent lack of biological response to such loadings: reservoirs tend to have greater water inflows than natural lakes and thus shorter water residence times, which flush the systems more thoroughly and limit the extent of, for example, algal growth. This is especially noticeable in reservoirs that have water residence times of less than two weeks, which is the typical period during which algal populations develop.
Less obvious characteristics include the gradation of water quality from the upper reaches of a reservoir to the lower reaches, or portion closest to the dam wall. This is especially pronounced in long sinuous reservoirs. A typical gradation in reservoir water quality is illustrated in Figure 1.2. This is in contrast to natural lakes which generally have a more rounded aspect and, hence, a more consistent water quality throughout. Such characteristics in reservoirs can be further modified by reservoir operations. For example, because reservoirs are constructed for specific purposes, provisions are generally made in their design for the withdrawal of water from the basin. In many cases, these draw-off points are located at or near the dam wall (for reasons of ease of construction etc.). Depending on the purpose of the reservoir, withdrawal of water has traditionally been from either the surface (if the purpose of the reservoir was to provide an added hydraulic head for hydropower purposes) or bottom (in most other cases) of the reservoir. This is in contrast to natural lakes where surface water overflow is the most common outlet for lake waters, and can significantly modify not only the thermal and chemical characteristics of the reservoir lake but also have severe repercussions downstream. Withdrawal of water from the surface of the reservoir, shown schematically in Figure 1.3 by arrow A, would result in the discharge of oxygenated, relatively warm water, rich in aquatic life. Withdrawal of water from the bottom of the reservoir (arrow B) would result in the discharge of deoxygenated, relatively cold water. Withdrawal of water from either source generally results in the discharge of water that has a lower suspended solids content than is typically present in the river, due to deposition in the delta (shown in Figure 1.3), leading to enhanced scouring of stream sediments downstream. In contrast, the withdrawal of water from the very lowest portion of the reservoir (through the outlet commonly known as the âscourâ valve, arrow C) can result in the release of substantial amounts of accumulated sediment, contaminants and oxygen-consuming substances into the downstream portions of the watershed with equally severe consequences.
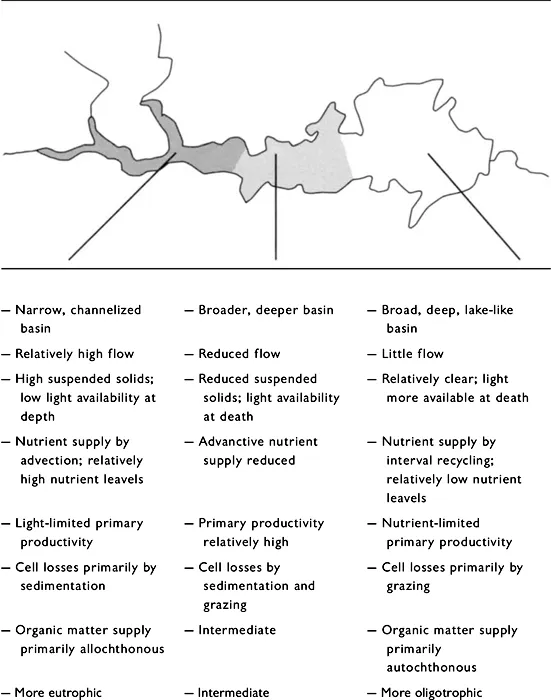
Figure 1.2 A typical trophic, or water quality, gradient observed in reservoirs (redrawn from Ryding and Rast 1989).
Figure 1.3 also illustrates a further characteristic of reservoirs, which is that the deepest portions of these artificial waterbodies tend to be located at one end of the system, in contrast to natural lakes where the deepest portions tend to be more centrally located (this characteristic is analogous to the locations of the waterbodies within their watersheds). The thermal stratification shown in Figure 1.3 is a common feature between lakes and reservoirs, but even that can be manipulated within reservoirs as a result of reservoir operations. When a reservoir is at full supply level, or its design depth, it may behave in a manner similar to a natural lake of the same size, mixing completely from top to bottom once (in the tropics) or twice (in the temperate zone) per year (in other words, the thermocline shown in Figure 1.3 is present for only part of the year, generally during summer). However, if the pool level, or water elevation, is reduced during the period(s) of annual stratification, the lake may become shallow enough to mix more frequently, resulting in a relatively quick change in water temperature and oxygen levels that can affect both aquatic life and water usage.
As noted, reservoirs are generally constructed for some purpose, often referred to as a beneficial use. These uses generally have associated water quality requirements. For example, water to be used for domestic consumption should have as low an algal content as possible and be free of contaminants injurious to the public health. This requirement will typically necessitate withdrawal of water from a depth below the surface, where algal growth is concentrated, but above the thermocline, below which contaminants such as iron and manganese (which impart an undesirable brownish colouration to the final water) occur. Similarly, water withdrawn for hydroelectric generating purposes should preferably be free of large objects, such as aquatic plants or debris that have accumulated behind the dam wall, and sufficiently well oxygenated (but not too well oxygenated) to prevent pitting of turbine blades. It is not unusual for the beneficial uses of a reservoir to change or be augmented during the life of the waterbody.

Figure 1.3 Diagrammatic representation of typical points of water withdrawal within a reservoir (redrawn from Chapman 1992).
1.4 Consequences of reservoirs
Thus far, this discussion has been confined to the definition of reservoirs and their distinguishing characteristics, i.e. those features that make reservoirs a distinct type of waterbody. Many of these characteristics have implications for the environment both within the reservoir and elsewhere in the watershed, both upstream and downstream of the impoundment. Some of these implications are a consequence of the age of the reservoir. As noted, reservoirs are constructed lakes having a predetermined life, usually about thirty to fifty years, but some have shorter lifetimes (for example, one South African reservoir was completely engulfed by sediment eroded from within its watershed within 10 years of the date of closure of the wall, although, the benefits of sediment retention were such that construction of the lake was economical), and some longer ones (many European and North American reservoirs/mill ponds are over a century old, while the Roman reservoirs in Spain, described above, are 1,800 years old).
The ageing process that occurs within reservoirs is both different from and similar to that in natural lakes. The ageing process differs from natural lakes in that it is typically shorter. As mentioned, reservoirs can be documented in historical time rather than geological time and segmented into distinct phases, the duration of which can be modified as part of the construction and operation of the reservoir. Figure 1.4 shows a typical example of the lake ageing process drawn from data on Lake Kariba, extending from Zimbabwe to Zambia. Phase I is the filling phase that follows closure of the dam wall. During this phase, organic matter present in the newly created lake basin rapidly decomposes, resulting in extensive deoxygenation and the release of a surge of nutrients into the overlying water. This results in an initial productivity peak or âtrophic surgeâ. The duration of this phase can be managed to a greater or lesser degree by clearing or partially clearing the lake basin of such material. For example, the period of âunbalanced eutrophyâ was much shorter for Kainji Lake, Nigeria, which was 70 per cent cleared, than for Lake Kariba, which was only 18 per cent cleared. Phase II and III represent a transition phase that can be described in terms of a vulnerable stability and secured stability. Phase II is distinguished from phase III by the dominance of riverine species, i.e. the lake retains many river-like characteristics, while phase III is dominated by lacustrine species, i.e. the lake takes on lake-like characteristics. Also during these phases, the trophic activity within the lake basin switches from a condition of internal nutrient supply, or from autochthonous sources, to one of external nutrient supply, where the reservoir effectively becomes a lake.
At this point, the lake ageing process mirrors that in natural lakes: over time, materials carried in from the watershed continue to accumulate in the lake basin until the depth of water is decreased by sedimentation, and decomposition processes and internal nutrient cycling again dominate. This process continues until the reservoir basin becomes a wetland, a marsh and, ultimately, a terrestrial system. As an aside, limnological studies of Spirit Lake, the natural lake destroyed and recreated by the Mount St Helens eruption in the USA, suggest that newly created natural lakes may also follow all four of these phases, undergoing an initial trophic surge, followed by a period of colonization and stabilization, and ultimate maturity.
Of particular concern during the initial phases of reservoir creation are the effects of inundation. Much knowledge concerning the impacts of inundation on the terrestrial environment was gathered during âOperation Noahâ, mounted as a wildlife rescue during the creation of Lake Kariba. Other rescues (e.g. at Lake Brokopondon, Surinam) have been launched since. These efforts have highlighted the tremendous disruption to animal migration patterns that occurs when a lake is created. In addition, follow-up studies conducted after the rescued wildlife is released suggest that even microscale differences in altitude, vegetation, and terrain can prove fatal. Little wildlife, in fact, has been successfully relocated as the result of such rescues. The obvious terrestrial impact, therefore, is the loss of endangered and threatened species from within the reservoir basin.

Figure 1.4 The ageing process as obse...
Table of contents
- Cover Page
- Title Page
- Copyright Page
- Contributors
- Foreword
- Preface
- Chapter 1: Reservoirs: environmental process, management and policy
- Chapter 2: Environmental indicators of healthy water resources
- Chapter 3: Water resources development and health: the policy perspective
- Chapter 4: Water resources development: policy perspectives of the Food and Agricultural Organization in relation to food security
- Chapter 5: Context and principles of environmental and health impact assessment
- Chapter 6: Health opportunity assessment in water resource development
- Chapter 7: Health opportunities in water resources development: a course promoting intersectoral collaboration
- Chapter 8: Ord River irrigation area: the effect of dam construction and irrigation on the incidence of Murray Valley encephalitis virus
- Chapter 9: Safe management of the Ross River dam, Northern Australia, in relation to recreation and health
- Chapter 10: Artificial wetlands and mosquito control in Australia
- Chapter 11: Environmental concerns and recreational development in relation to public health issues associated with water resource projects of the Tennessee Valley Authority
- Chapter 12: The Tennessee Valley Authority programme to control mosquitoes in fresh water impoundments and wetlands
- Chapter 13: Aquaculture, anophelines and environmental solutions from Indonesia
- Chapter 14: Socioeconomic and health impacts of water resources development in Thailand
- Chapter 15: Urban water resource problems in vector-borne disease with special reference to dengue viruses