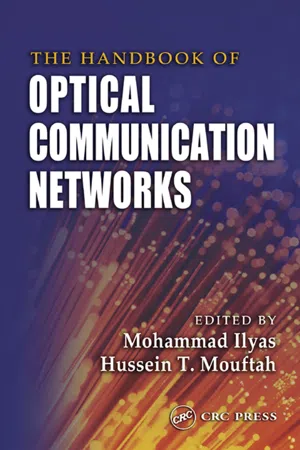
This is a test
- 488 pages
- English
- ePUB (mobile friendly)
- Available on iOS & Android
eBook - ePub
The Handbook of Optical Communication Networks
Book details
Book preview
Table of contents
Citations
About This Book
The Internet revolution. Once, the public was delighted with 14.4 modem access and fascinated by low-tech Web site content. But not for long. Technology has raced to keep up with users' calls for high-speed facilities and advanced applications. With the development of high-speed transmission media and the availability of high-speed hardware, we are
Frequently asked questions
At the moment all of our mobile-responsive ePub books are available to download via the app. Most of our PDFs are also available to download and we're working on making the final remaining ones downloadable now. Learn more here.
Both plans give you full access to the library and all of Perlegoâs features. The only differences are the price and subscription period: With the annual plan youâll save around 30% compared to 12 months on the monthly plan.
We are an online textbook subscription service, where you can get access to an entire online library for less than the price of a single book per month. With over 1 million books across 1000+ topics, weâve got you covered! Learn more here.
Look out for the read-aloud symbol on your next book to see if you can listen to it. The read-aloud tool reads text aloud for you, highlighting the text as it is being read. You can pause it, speed it up and slow it down. Learn more here.
Yes, you can access The Handbook of Optical Communication Networks by Mohammad Ilyas,Hussein T. Mouftah in PDF and/or ePUB format, as well as other popular books in Physical Sciences & Optics & Light. We have over one million books available in our catalogue for you to explore.
Information
chapter one
Overview of optical communication networks: Current and future trends *
AysegĂŒl Gençata
Istanbul Technical University
Narendra Singhal
University of California, Davis
Biswanath Mukherjee
University of California, Davis
1.1 Introduction
The focus of this chapter is to present technological advances, promising architectures, and exciting research issues in designing and operating next-generation optical wavelength-division multiplexing (WDM) networks, which are scalable and flexible. We discuss important building blocks of optical WDM networks and overview access, metropolitan, and long-haul networks separately. Special attention has been paid to the long-haul network because there is a tremendous need to develop new intelligent algorithms and approaches to efficiently design and operate these wide-area-optical-mesh networks built on new emerging technologies. We present several research topics including routing and wavelength assignment, fault management, multicasting, traffic grooming, optical packet switching, and various connection-management problems. The Internet is developing rapidly with the ultimate goal being to provide us with easy and fast access to any desired information from any corner of the world. Information exchange (or telecommunications) technology, which has been evolving continuously since the telephone was invented, is still striving to meet the usersâ demands for higher bandwidth. This demand is attributed to the growing popularity of bandwidth-intensive networking applications, such as data browsing on the World Wide Web (WWW), Java applications, video conferencing, interactive distance learning, on-line games, etc. Figure 1.1 plots the past and projected growth of data and voice traffic as reported by most telecom carriers. 1 It shows that, while voice traffic continues to experience a healthy growth of approximately 7% per year, data traffic has been growing much faster. To support this exponential growth in the user data traffic, there is a strong need for highbandwidth network facilities, whose capabilities are much beyond those of current highspeed networks such as asynchronous transfer mode (ATM), SONET/SDH* etc. 2
Fiber-optic technology can meet the previously mentioned need because of its potentially limitless capabilities: 3 huge bandwidth (nearly 50 terabits per second [Tbps] for single-mode fiber), low signal attenuation (as low as 0.2 dB/km), low signal distortion, low power requirement, low material usage, small space requirement, and low cost. Given that a single-mode fiberâs potential bandwidth is nearly three orders of magnitude higher than electronic data rates of a few tens of gigabits per second (Gbps), we need to tap into this huge optic-electronic bandwidth mismatch. Because the maximum rate at which an end userâwhich can be a workstation or a gateway that interfaces with lower-speed sub-networksâcan access the network is limited by electronic speed, concurrency among multiple user transmissions should be introduced to exploit the fiberâs huge bandwidth.
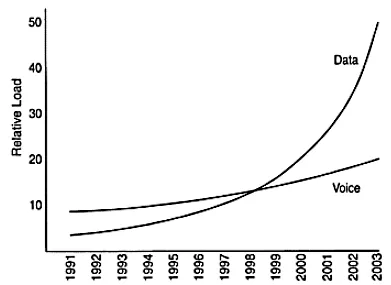
Figure 1.1 Past and projected future growth of data and voice traffic.
WDM is a favorite multiplexing technology in optical communication networks because it supports a cost-effective method to provide concurrency among multiple transmissions in the wavelength domain. Several communication channels, each carried by a different wavelength, are multiplexed into a single fiber strand at one end and demultiplexed at the other end, thereby enabling multiple simultaneous transmissions. Each communication channel (wavelength) can operate at any electronic processing speed (e.g., OC-192 or OC-768).* For example, a fiber strand that supports 160 communication channels (i.e., 160 wavelengths, each operating at 40 Gbps) would yield an aggregate capacity of 6.4 Tbps.
Todayâs telecom network can be considered to consist of three sub-networks: access (spanning about 1 to 10 km), metropolitan (covering about 10 to 100 km), and long haul (extending to 100s or 1000s of km) (see Figure 1.2); and fiber is being extensively deployed in all three sub-networks. Typically, the network topology for access can be a star, a bus, or a ring; for metro a ring; and for long haul a mesh. Each of these subnetworks has a different set of functions to perform; hence, each has a different set of challenges, technological requirements, and research problems. For example, for the long-haul network, carriers are more concerned with capacity, protection, and restoration, while for the metro or access network, carriers are more concerned with service provisioning/monitoring, flexibility, etc.
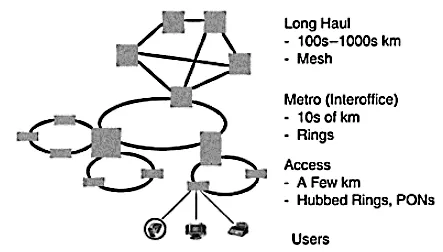
Figure 1.2 Telecom network overview.
The focus of this chapter is to present technological advances, promising architectures, and exciting research issues in designing and operating next-generation optical WDM networks that are scalable and flexible. The next section provides a brief discussion on the important building blocks of optical WDM networks. This is followed by an overview of access, metropolitan, and long-haul networks separately. Special attention has been paid to the long-haul network because there is a tremendous need to develop new intelligent algorithms and approaches to efficiently design and operate these wide-areaoptical- mesh networks built on new emerging technologies. We present several research topics including routing and wavelength assignment (RWA), fault management, multicasting, traffic grooming, optical packet switching, and various connectionmanagement problems.
1.2 Enabling WDM technologies
An important factor to consider in the design of a WDM network is the number of wavelengths to use. The maximum number of wavelengths is limited by optical device technology and is affected primarily by the total available bandwidth or spectral range of the components (including the fiber) and the spacing between channels. Conventional fibers have a low attenuation region between 1335 and 1625 nm with a âwater-peak windowâ at 1385 nm. New âall-waveâ fibers do not have this water peak and hence can use a larger spectrum (see Figure 1.3). Channel spacing itself is affected by several factors such as the channel bit rates, optical power budget, non-linearities in the fiber, and the resolution of transmitters and receivers. In dense wavelength-division multiplexing (DWDM), a large number of wavelengths (>160) is packed densely into the fiber with small channel spacing. An alternative WDM technology with a smaller number of wavelengths (< 10), larger channel width, larger channel spacing, and much lower cost is termed as coarse WDM (CDWM).

Figure 1.3 Low-attenuation region of all-wave fiber vs. conventional fiber.
Although new approaches and technologies are constantly under development, this section highlights some of the emerging and novel technologies that can revolutionize the design and effectiveness of WDM networks. Optical components employed in building a typical point-to-point optical WDM transmission system are depicted in Figure 1.4. Several optical signals sent by transmitters (lasers) are coupled together using a (wavelength) multiplexer into a fiber. Signals are amplified, when necessary, using amplifiers such as erbium-doped fiber amplifiers (EDFAs) to compensate for signal attenuation. At intermediate nodes, these signals can be dropped and new signals can be added using optical add drop multiplexers (OADMs). At the receiving end, a (wavelength) de-multiplexer is used to segregate the individual wavelengths arriving on the fiber, which are then fed into the receivers (filters).
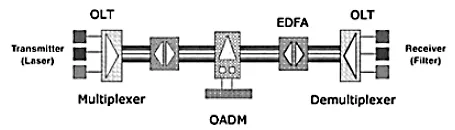
Figure 1.4 A typical point-to-point optical fiber communication link.
The EDFAs with gain spectrum of 30 to 40 nm each (typically in the 1530 to 1560 nm range; see Figure 1.4) can be interconnected to broaden their gain bandwidth. This âamplifier circuitâ is referred to as an ultrawide-band EDFA, which can fully exploit the expanded low-attenuation region of the new âall-wave fiberâ (see Figure 1.4).
OADMsâalso referred to as wavelength add-drop multiplexers (WADMs)âare employed to take in (add) and take out (drop) individual wavelengths from an optical fiber completely in the optical domain (i.e., without any conversion of the optical signal into electronic domain). OADMs, which can add and drop a specific predefined channel (or a group of channels), are said to be fixed-tuned (or static) and the technology for manufacturing them is mature. Reconfigurable OADMs (ROADMs), with add-drop wavelengths that can be controlled by an external stimulus (e.g., by software) are said to be tunable (or dynamic). ROADMs are more powerful because they can adapt to the fluctuating traffic demand but the technology for building ROADMs is still in nascent stage.
When the network topology is a mesh, where nodes are interconnected by fibers to form an arbitrary graph, an additional fiber interconnection device is needed to route the signals from an input port to the desired output port. These devices are called optical crossconnects (OXCs). They can either be transparent (to bit rates and signal formats) in which signals are switched ail-optically or be opaque in which incoming signals are converted from optical to electronic domain and switched electronically. A possible architecture for a transparent OXC is presented in Figure 1.5, where all signals on a particular wavelength (e.g., λ1) arriving on M input fibers are switched separately by a wavelength-specific MxM switch. As more and more wavelengths are packed into a fiber, the size of the OXCs is expected to increase. Among several technologies (e.g., bubble, liquid crystal, thermo-optic, holographic, electro-optic, LiNbO3, etc.) used for building all-optical OXCs, MEMS (micro-electro-mechanical-systems) based OXCs are becoming popular because of their compact design, low power consumption, and promise for high port count. 4 For details on emerging MEMS-based OXC architectures, please refer to Reference 5.
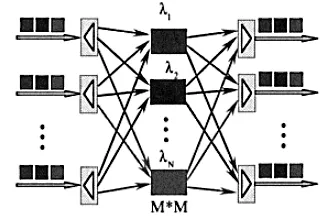
Figure 1.5 An optical crossconnect (OXC) of size NMĂNM (N is the number of wavelengths; M is the number of incoming/outgoing fibers).
1.3 Access networks
The access network connects the subscribers (home or business) to the service providers; in other words, it serves as the âlast mileâ (as well as the âfirst mileâ) of the information flow. To meet the growing traffic demand, service providers expend most of their effort on increasing the bandwidth on their backbone network. But little has changed in the access network. It is now the general opinion that the last mile has become a bottleneck in todayâs network infrastructure. 6 Optical technology is a promising candidate for solving the bandwidth problem in access networks because it can provide at least 10 to 100 times more bandwidth over a larger coverage area. The next wave in access network deployment will bring the fiber to the building (FTTB) or to the home (FTTH), enabling Gbps speeds at costs comparable to other technologies such as digital subscriber line (DSL) and hybrid fiber coax (HFC). 6
Three optical technologies are promising candidates for the next-generation access networks: point-to-point topologies, passive optical networks, and free-space optics.
1.3.1 Point-to-point topologies
Point-to-point dedicated fiber links can connect each subscriber to the telecom central office (CO), as illustrated in Figure 1.6a. This architecture is simple but expensive due to the extensive fiber deployment. An alternative approach is to use an active star topology, where a curb switch is placed close to the subscribers to multiplex/de-multiplex signals between the subscribers and the CO. This alternative in Figure 1.6b is more cost effective in terms of deployed fiber. A disadvantage of this approach i...
Table of contents
- Cover Page
- Title Page
- Copyright Page
- Preface
- About the Editors
- Contributors
- chapter one Overview of optical communication networks: Current and future trends
- chapter two Evolution of optical networks architecture
- chapter three Design aspects of optical communication networks
- chapter four Evolution to an optical broadband services network
- chapter five Multiprotocol label switching
- chapter six Dynamic synchronous transfer mode
- chapter seven A survey on fair bandwidth allocation for multicast over the Internet
- chapter eight Emerging optical network management
- chapter nine Optical network resource management and allocation
- chapter ten Real-time provisioning of optical communication networks
- chapter eleven Routing and wavelength assignment with multi-granularity traffic in optical networks
- chapter twelve Adaptive routing and wavelength assignment in all-optical networks: the role of wavelength conversion and virtual circuit deflection
- chapter thirteen Connection management in wavelength-routed all-optical networks
- chapter fourteen A novel distributed protocol for path selection in dynamic wavelength-routed WDM networks
- chapter fifteen Distributed lightpaih control for wavelength-routed WDM networks
- chapter sixteen Recent advances in dynamic lightpath restoration in WDM mesh networks
- chapter seventeen Restoration in optical WDM mesh networks
- chapter eighteen Shared alternate-path protection with multiple criteria in all-optical wavelength-routed WDM networks
- chapter nineteen Optical transport networks: A physical layer perspective
- chapter twenty Fiber optic sensors
- chapter twenty-one Wavelength converters
- Glossary