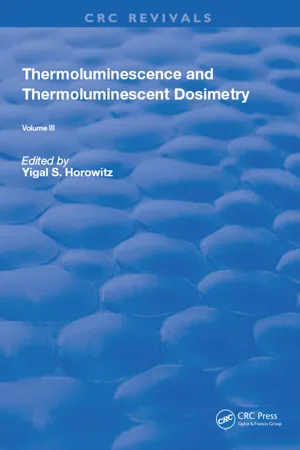
This is a test
- 216 pages
- English
- ePUB (mobile friendly)
- Available on iOS & Android
eBook - ePub
Thermoluminescence and Thermoluminescent Dosimetry
Book details
Book preview
Table of contents
Citations
About This Book
First Published in 1984, this set offers a comprehensive insight into thermolumiscence. Carefully compiled and filled with a vast repertoire of notes, diagrams, and references this book serves as a useful reference for radiobiologists and physicists and other practitioners in their respective fields.
Frequently asked questions
At the moment all of our mobile-responsive ePub books are available to download via the app. Most of our PDFs are also available to download and we're working on making the final remaining ones downloadable now. Learn more here.
Both plans give you full access to the library and all of Perlego’s features. The only differences are the price and subscription period: With the annual plan you’ll save around 30% compared to 12 months on the monthly plan.
We are an online textbook subscription service, where you can get access to an entire online library for less than the price of a single book per month. With over 1 million books across 1000+ topics, we’ve got you covered! Learn more here.
Look out for the read-aloud symbol on your next book to see if you can listen to it. The read-aloud tool reads text aloud for you, highlighting the text as it is being read. You can pause it, speed it up and slow it down. Learn more here.
Yes, you can access Thermoluminescence and Thermoluminescent Dosimetry by Yigal S. Horowitz in PDF and/or ePUB format, as well as other popular books in Sciences physiques & Chimie analytique. We have over one million books available in our catalogue for you to explore.
Information
Chapter 1
TL READOUT INSTRUMENTATION
Per Spanne
TABLE OF CONTENTS
I. | Introduction | ||
II. | Signal-to-Noise Ratios in TL Measurements | ||
III. | Dosimeter Heating Systems A. General Aspects B. Ohmic Heating 1. Heating Elements with Varying Temperature 2. Heating Elements with Constant Temperature C. Hot Gas D. Optical Heating E. RF Heating F. Internally Heated Dosimeters G. String Dosimeter Heating | ||
IV. | Light Collection Unit A. Readout Chamber B. Optical Filters 1. Incandescent Light Emission 2. Reduction of Nonradiation-Induced TL C. Light Collection on the Photoelectric Detector | ||
V. | Light-Measuring Systems A. Introduction B. PM Tubes 1. General Principle 2. Quantum Efficiency 3. Collection Efficiency 4. Dynodes and the Resistor Chain 5. Electric and Magnetic Shields C. PM Tube Dark Signal 1. General Aspects 2. Thermionic and Field Emission 3. Interactions of Ionizing Radiation 4. Photocathode Disturbance 5. Ion Pulses 6. Leakage Currents 7. Other Effects D. Signal Registration 1. General Aspects 2. Glow Curves 3. Charge-Measuring Techniques 4. Photon Counting Techniques 5. Optimizing the Potential Difference Across the PM Tube 6. Comparison of Photon Counting and Charge Integration E. Other Photodetectors F. Reference Light Sources G. Instruments for Measurements of TL Spectra | ||
VI. | Aspects of Instruments for TL Dating | ||
VII. | Calibration Phantom | ||
VIII. | Aspects of Annealing Ovens | ||
References |
I. INTRODUCTION
Instrumentation for the study and quantification of the thermoluminescence (TL) from a material is composed of two main parts: a heating unit and a light-measuring unit. The first readout device, used by Sir Robert Boyle to observe TL from diamonds,1 consisted of his own body heat and the naked eye. Far more sophisticated readout techniques have been developed since then.
The requirements on a TL readout instrument are strongly dependent on the purpose of the TL measurement, e.g., for TL materials research it is essential that the temperature of the sample and that of the heating device closely correspond to enable accurate determination of the sample temperature during readout. This is usually achieved with slow linear heating rates, often less than 1°C/sec. A slow heating rate also usually results in optimum glow peak resolution. On the other hand, for many radiation dosimetry measurements, e.g., routine personnel monitoring of ionizing radiation, it is important that the dosimeter heating is sufficiently rapid to enable the evaluation of many dosimeters in a reasonable time and that the background signals from the readout unit are kept to a minimum.
There exist many reports describing different TL readout instruments designed for various purposes. Some of these are available commercially. In this chapter on TL instrumentation we will, after some introductory remarks on signal-to-noise ratios in TL measurements, treat the principles of different heating systems and light measuring techniques used for investigations of TL materials and TL dosimetry. Finally, some aspects of the auxiliary equipment necessary for reliable TL dosimetry will be discussed.
II. SIGNAL-TO-NOISE RATIOS IN TL MEASUREMENTS
The measurement of TL light emission is always accompanied by systematic and statistical uncertainties. An example of a systematic uncertainty is the error introduced if the dark current of the photomultiplier increases with time during a measuring series because of heating of the photomultiplier photocathode. Statistical uncertainties appear among other reasons because of the quantum nature of light. In specifying the performance of a TL readout system or a TL dosimetry system, it is therefore necessary to specify both the statistical and systematic uncertainties.2 In the performance, testing, and procedural specifications for thermoluminescence dosimetry in environmental applications, issued by the American National Standards Institute (ANSI),3 no distinction is made between the two types of uncertainties, rendering it impossible to evaluate some of the performance requirements.
As a measure of the statistical uncertainty (precision) in a TL measurement, the signal-to-noise ratio defined as E(S)/σ or the relative standard deviation which is the inverted value of the signal-to-noise ratio can be used. S stands for the TL signal, E(S) for the expectation value of S, and a for the standard deviation of the measured TL signal, a is a measure of the random variations in the measured signal and is often referred to as the noise in the measured signal. In some papers noise has been used to denote the total background signal. This usage should be discouraged since it is the random fluctuations in the signals, not their absolute values that affect the precision in the measurements. A noiseless background signal of whatever magnitude does not constitute a problem since it can be subtracted so that it does not disturb the measurement. In this work noise is used exclusively to denote the random variations in a signal. Whether one uses the signal-to-noise ratio or its inverted value, the relative standard deviation, to specify the statistical performance of a TLD system really does not matter. The only advantage with the signal-to-noise ratio is that it increases as the precision increases.
The TL signal, S, is determined experimentally as the difference between signals in two separate measurements: one measuring the radiation-induced TL plus background signals, the other measuring only the background signals. Sometimes the background signals are measured several times to improve the precision. In this case the signal-to-noise ratio for a measurement can be written as
(1) |
where S is the TL signal; B is all background signals except DP, where DP is the background signal caused by the photoelectric device used for light detection; σS, σB, and σDP are the standard deviations of S, B, and DP, respectively; and h is the number of measurements carried out in estimating the total background signal (B + DP).
This equation is valid when S, B, and DP are independent stochastic variables. The factor (1 + 1/h) appears in Equation 1 since the background signals B and DP have to be determined separately under the same conditions as the total signal. If the conditions change between the measurement of the total signal and the background signals, a systematic error is introduced. The signal-to-noise ratio varies slowly with h when h is larger than 5, e.g., it increases by only 5% when h is changed from 5 to 10. This slow dependence on h is due to the fact that Equation 1 is valid when the TL signal is measured with a single dosimeter or a single sample of TL material. It is possible to use several dosimeters in order to increase the signal-to-noise ratio. The optimization problem is then similar to that of dividing a given time interval between signal and background measurements treated in detail by Young.4
Analyzing Equation 1 shows that each of its constituents depends on several parameters, some of them interrelated in such a complex way that it is impossible to determine unambiguously the best way for obtaining the optimum performance of a TLD system. However, it turns out that the quantum efficiency of the photoelectric device and the fraction of photons transmitted through the optical filter are the only variables that can selectively decrease the background signals in such a way that the noise in the background signal, σB, is decreased by a larger factor than the TL signal, with the net effect that the signal-to-noise ratio increases although the TL signal decreases.5 A consequence of this is that it is practical to proceed in three steps5 when optimizing the signal-to-noise ratio for a TLD system, i.e., first optimize the light-collecting and light-measuring equipment exclusive of the optical filter, then the dosimeter sensitivity, and finally the signal-to-noise ratio by reduction and control of background signals.
With respect to the systematic uncertainties caused by the readout equipment, the general guideline to follow in the optimization is to minimize all kinds of drift in the equipment. In this connection one should be aware that drift, e.g., in the light sensitivity of the photoelectric device, certainly affects an experimentally estimated signal-to-noise ratio when the estimate is determined from repeated measurements. However, this does not imply that an estimate of the signal-to-noise ratio alone is sufficient to characterize the performance of a TLD system.
An experimentally estimated signal-to-noise ratio is a meaningful measure of the statistical uncertainty only when the drift in the readout equipment is small compared to the statistical uncertainty.
III. DOSIMETER HEATING SYSTEMS
A. General Aspects
The heating system in a TL readout instrument consists in principle of two parts: a heat supplying medium or device and electronics for the control of the temperature. Two common examples of the former are a resistively heated metal planchet and a jet of hot gas. The temperature control unit, whose general purpose is to produce a reproducible heating cycle (by heating cycle is meant the variation of the temperature with time during readout) may be more or less complicated according to the application in which the TL reader is used. In a simple case it just consists of a transformer that generates an alternating current for ohmic heating of a metal planchet. In more sophisticated readout instruments, a temperature-measuring device is connected to a feedback circuit with programmable reference ramps and plateaux that allows a wide variation of usable heating cycles. By using a curve follower to generate the reference ramps, any heating cycle within the limits of the heat capacity of the heating device can be implemented simply by drawing it on a piece of paper.5
One of the most important requirements on a readout instrument for TL measurements is that the heating of the material under study should be reproducible. This is so because for many materials the amount of radiation-induced TL ...
Table of contents
- Cover
- Title Page
- Copyright Page
- Preface
- Editor
- Dedication
- Acknowledgement
- Contributors
- Thermo Luminescence and Thermoluminescent Dosimetry
- Table of Contents
- Chapter 1 TL Readout Instrumentation
- Chapter 2 Clinical Applications of Thermoluminescent Dosimetry
- Chapter 3 Thermoluminescence Applied to Age Determination in Archaeology and Geology
- Index