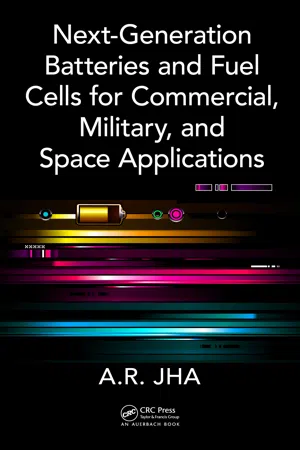
eBook - ePub
Next-Generation Batteries and Fuel Cells for Commercial, Military, and Space Applications
This is a test
- 416 pages
- English
- ePUB (mobile friendly)
- Available on iOS & Android
eBook - ePub
Next-Generation Batteries and Fuel Cells for Commercial, Military, and Space Applications
Book details
Book preview
Table of contents
Citations
About This Book
Distilling complex theoretical physical concepts into an understandable technical framework, Next-Generation Batteries and Fuel Cells for Commercial, Military, and Space Applications describes primary and secondary (rechargeable) batteries for various commercial, military, spacecraft, and satellite applications for covert communications, surveillan
Frequently asked questions
At the moment all of our mobile-responsive ePub books are available to download via the app. Most of our PDFs are also available to download and we're working on making the final remaining ones downloadable now. Learn more here.
Both plans give you full access to the library and all of Perlegoās features. The only differences are the price and subscription period: With the annual plan youāll save around 30% compared to 12 months on the monthly plan.
We are an online textbook subscription service, where you can get access to an entire online library for less than the price of a single book per month. With over 1 million books across 1000+ topics, weāve got you covered! Learn more here.
Look out for the read-aloud symbol on your next book to see if you can listen to it. The read-aloud tool reads text aloud for you, highlighting the text as it is being read. You can pause it, speed it up and slow it down. Learn more here.
Yes, you can access Next-Generation Batteries and Fuel Cells for Commercial, Military, and Space Applications by A.R. Jha in PDF and/or ePUB format, as well as other popular books in Computer Science & Information Technology. We have over one million books available in our catalogue for you to explore.
Information
Chapter 1

Current Status of Rechargeable Batteries and Fuel Cells

1.1 Rechargeable Batteries
The need to eliminate dependency on costly foreign oil and the adverse effects of harmful gases on health has compelled energy experts to search for alternate battery technologies for possible deployment in electric vehicles (EVs) and hybrid electric vehicles (HEVs) desired for transportation. Energy experts and transportation consultants have recommended the use of high-capacity, lithium-ion rechargeable batteries for electric and hybrid vehicles. Battery designers indicate that Nissan Motor Company and Sony Corporation have deployed rechargeable Li battery packs in their electric and hybrid vehicles. General Motors and Siemens Company have invested significant money in research and development for fuel cells best suited for trucks and buses. Battery requirements for zero-emission vehicles (ZEVs) will be more stringent. Sealed nickel-cadmium (Ni-Cd) batteries are currently used in commercial (MD-80, DC-9, and Boeing 777) and military aircrafts (F-16, F-18, and E-8). To meet high longevity and reliability requirements, vented Ni-Cd batteries previously used by various commercial and military aircraft should be replaced by high-performance, maintenance-free sealed Ni-Cd rechargeable batteries. Both sealed lead-acid (Pb-acid) and vented Ni-Cd rechargeable batteries are widely deployed by the commercial and military aircraft to meet improved efficiency, reliability, longevity, and output power requirements.
Requirements of next-generation batteries will focus on low cost, light weight, compact packaging, portability, and longevity exceeding 15 years. Rechargeable batteries will be found most suitable for battlefield weapons, communication satellites, space reconnaissance and surveillance systems, underwater tracking sensors, and a host of several applications. Note that high-capacity batteries are best suited for commercial and military aircraft, helicopters, drones, hybrid vehicles, space sensors, and battlefield weapons, whereas low-power rechargeable batteries are widely deployed by the cell phones, laptops, medical devices, computers, and host of other electronic and digital devices. The integration of microminiaturization technology [1] involving the microelectrical mechanical system (MEMS) and nanotechnology techniques will be given serious consideration in the design and development of next-generation rechargeable batteries to meet the stringent performance specifications, including reliability, portability, longevity, and compact packaging.
Current aggressive research and development activities are directed toward improving the design of nickel-zinc (Ni-Zn) rechargeable batteries, which have a great promise to offer the lowest transportation costs between $0.03 and $0.44 per kilometer (km). The design and development activities must also focus on critical electrical parameters such as the state of charge (SOC), thermal runaway, charge and discharge rates, discharge cutoff detection, and correlation between the SOC and open-circuit voltage (OCV). For battlefield rechargeable batteries, weight, size, cost, reliability, and longevity are the most essential design requirements.
1.2 Fundamental Aspects of a Rechargeable Battery
A battery consists of one or more voltaic cells. Each voltaic cell consists of two half-cells. Negatively charged anions migrate to the anode electrode (negative electrode) in one half-cell, while the positively charged cations migrate to the cathode (positive electrode) in the other half-cell. The electrodes are separated by an electrolyte medium, which is ionized to create the anions and cations and to permit the movement of those ions.
In some battery design, the half-cells have different electrolytes. In such cases, a separator prevents mixing, but the ions can squeeze through in many cells, from carbon-zinc (C-Zn) through Ni-Cd and Li. The most critical performance parameters for various rechargeable batteries, namely the energy density (expressed in watt-hour per kilogram, or Wh/kg) and the power density (expressed in watt-hour per liter, or Wh/L), are shown in Figure 1.1 along the y-axis and x-axis, respectively. The electrolyte medium is merely a buffer for an ion flow between the electrodes. However, in the case of Pb-acid cells, which are widely used in automobiles, the electrolyte is a part of the electrochemical reaction.
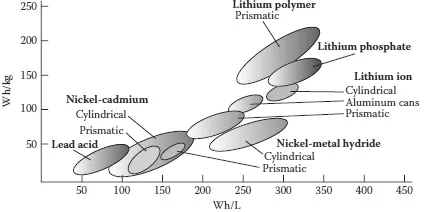

Figure 1.1 Specific power (Wh/kg) and energy density (Wh/L) for various rechargeable batteries.
The OCV of a charged battery is the electromotive force (EMF) of a cell, which comes from the difference between the reduction potentials of the reactions in the half-cells. During the discharge phase, the battery converts the heat energy that would be released during the chemical reaction between the cathode and anode terminals. This heat energy is converted into electrical energy.
Real-life batteries exhibit an equivalent series resistance (ESR) internally, which drops some of the OCV when the battery is used in an external circuit or load. The ESR increases as the battery discharges or provides electrical energy to an external load, and the battery terminal voltage will drop under load conditions. Typical charge and discharge characteristics of thin-film batteries are displayed in Figure 1.2. In addition, the batteries tend to discharge during the dormant period. Some batteries tend to discharge more compared with others depending of their types. Studies performed by the author have indicated that Ni-Cd and nickel-metal-hydride (Ni-MH) [2] batteries will discharge at an approximate rate of 20% per month compared with Li batteries with 5 to 10% per month, Pb-acid batteries with 3 to 4% per month, and alkaline batteries with less than 0.3% per month. This clearly states that alkaline batteries suffer from lowest self-discharge rates during the dormant periods.
Rechargeable batteries are inherently rechargeable by reversing the chemical reactions that took place during the discharge. Charging is a classic redox chemical process. The negative material is reduced, consuming electrons, and the positive material is oxidized and the electrons are produced. Regardless of the battery type, the output energy level and the power density are the key battery characteristics, and both are expressed in per unit volume and mass, respectively.
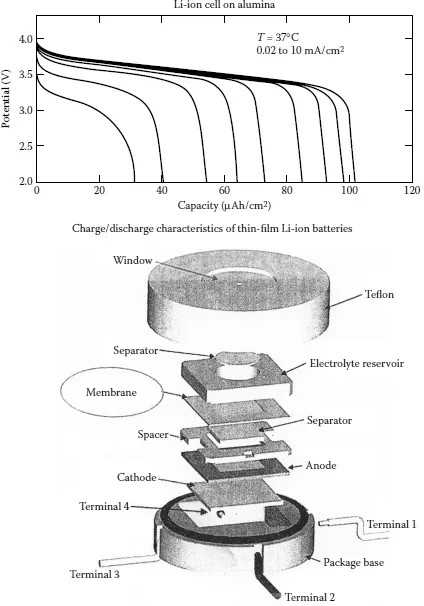

Figure 1.2 Architecture of a TF superhydrophobic nanostructured surface made from nanotubes.
1.2.1 Critical Performance Characteristics of Rechargeable Batteries
Two of the most critical performance characteristics of a rechargeable battery are the energy density (Wh/L) and the specific energy (Wh/kg). From these parameters, one can estimate the energy available from 1 kg of fuel and from one volume of fuel. Studies by the author have indicated that there are several types of rechargeable batteries in the current market. One can select a right battery for his specific applications [3]. Specific energy or energy density and the power density curves for various rechargeable batteries are shown in Figure 1.1. Estimated performance characteristics of various rechargeable batteries are summarized in Table 1.1.
Table 1.1 Performance Characteristics of Various Rechargeable Batteries
Battery Type and Classification | Specific Energy (Wh/kg) | Energy Density (Wh/L) |
Lead-acid | 25 | 70 |
Prismatic batteries | ||
Type 1 | 25 | 125 |
Type 2 | 40 | 160 |
Type | 72 | 215 |
Nickel-cadmium | 35 | 240 |
Nickel-metal-hydride | 62 | 260 |
Lithium-ion | 120 | 300 |
Lithium-phosphate | 160 | 290 |
Lithium-polymer (prismatic) | 188 | 294 |
These estimated values are batteries developed five years ago and are accurate within ±5%. The same batteries are now improved by 5 to 10%. Reliability, efficiency, and longevity of these batteries will be specified in subsequent chapters.
1.2.2 Capabilities of Widely Used Rechargeable Batteries in Commercial Applications
Specific energy (Wh/kg), energy density (Wh/L), longevity (operating hours), and self-discharge (percent per month) and specific power (W/kg) are given serious consideration in the selection of rechargeable power batteries for commercial applications. Performance characteristics of commercial batteries are summarized in Table 1.2.
Data on the popular Duracell alkaline cell are included just to put rechargeable batteries in proper perspective. Cycle life is strictly dependent on how the battery is treated or used. In the case of Li cells, the anode voltage drops from 4 volt to 3 volt in a linearly fashion. Ni-MH batteries are commercially made by Toshiba and could suffer from memory loss. Zinc-air cells were initially deployed as prototype batteries for EVs and HEVs. The performance data summarized in the Table 1.2 are not obtained under identical operating conditions for all cell types.
Table 1.2 Performance of Widely Used Batteries for Commercial Use
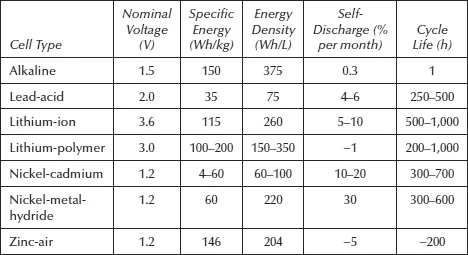
1.2.3 Recycling of Batteries
At the end of a batteryās useful life, its disposal becomes a critical issue to save the environment from the toxic effects of used batteries. Toxic effects from lead and lithium contents in batteries must be eliminated for environmental safety. In a recycling plant, as the spent batteries are dumped onto a conveyor belt, molten lead is converted into āingotsā called āpigsā and āhogs,ā and the operator guides the placement of recycled lead hogs into a separate container. In case of used lithium batteries, the batteries are disposed and stored in containers that can be buried underground to avoid the toxic leakage from the lithium.
Portability of electricity has become a part of daily living. Batteries are widely used by portable electric and electronic devices, such as telephones, computers, radios, compact disks, tape recorders, cordless tools, and even the electronic cars. But at the end of life, these batteries can come back to haunt us. A primary battery used for a flashlight lasts for a single life cycle, whereas in the case of a rechargeable battery, the battery can be recharged for thousands of cycles. From an environmental viewpoint, a rechargeable battery that is classified as a secondary battery is preferred over a primary battery in terms of material savings. Therefore, a single rechargeable battery is functionally equivalent to dozens of primary cells and saves recycling costs [4] involving hundreds of primary batteries. In addition, the life-cycle cost is not the slightest concern of a customer.
Worldwide, hundreds of millions of large batteries and millions of small batteries, containing tons of toxic and hazardous materials, are produced and used each year. Until recently, most of them were simply discarded. But because of the latest environmental laws, the Pb-acid and industrial Ni-Cd batteries are systematically collected for the sake of recycling their toxic and hazardous materials. Requirements for battery recycling vary from country to country, with a clear trend toward stricter controls on the requirements and disposal options. More sweeping regulations are under active consideration by the United States, Germany, France, and other European countries. A few decades back the European Union drafted a directive that would require recycling of at least 80% of all industrial and automobile rechargeable batteries. Recycling requirements are dependent on the materials used in the manufacturing of the rechargeable batteries. Only a fraction of battery materials can be recovered, which depends on three distinct factors:



For Pb-acid batteries, the return rate in the battery is more than 95%, the recoverable lead in the battery mass is about 60%, and the efficiency of a secondary smelter is roughly 95%. Considering all these factors, the material recycle fraction of the battery mass would be about 54%.
1.2.3.1 Toxicity of Materials Used in Manufacturing Rechargeable Batteries
Toxicity of some materials used in the design and development of rechargeable batte...
Table of contents
- Cover
- Title Page
- Copyright
- Dedication
- Contents
- Foreword
- Preface
- Author
- 1 Current Status of Rechargeable Batteries and Fuel Cells
- 2 Batteries for Aerospace and Communications Satellites
- 3 Fuel Cell Technology
- 4 Batteries for Electric and Hybrid Vehicles
- 5 Low-Power Rechargeable Batteries for Commercial, Space, and Medical Applications
- 6 Rechargeable Batteries for Military Applications
- 7 Batteries and Fuel Cells for Aerospace and Satellite System Applications
- 8 Low-Power Batteries and Their Applications
- Index