
eBook - ePub
To See But Not To See: A Case Study Of Visual Agnosia
This is a test
- 124 pages
- English
- ePUB (mobile friendly)
- Available on iOS & Android
eBook - ePub
To See But Not To See: A Case Study Of Visual Agnosia
Book details
Book preview
Table of contents
Citations
About This Book
Brain damage may sometimes cause specific impairments in human behaviour. One rare impairment is the failure to recognize everyday objects by sight, a problem which is termed "visual agnosia". In this book, the authors discuss the case of a patient,
Frequently asked questions
At the moment all of our mobile-responsive ePub books are available to download via the app. Most of our PDFs are also available to download and we're working on making the final remaining ones downloadable now. Learn more here.
Both plans give you full access to the library and all of Perlegoâs features. The only differences are the price and subscription period: With the annual plan youâll save around 30% compared to 12 months on the monthly plan.
We are an online textbook subscription service, where you can get access to an entire online library for less than the price of a single book per month. With over 1 million books across 1000+ topics, weâve got you covered! Learn more here.
Look out for the read-aloud symbol on your next book to see if you can listen to it. The read-aloud tool reads text aloud for you, highlighting the text as it is being read. You can pause it, speed it up and slow it down. Learn more here.
Yes, you can access To See But Not To See: A Case Study Of Visual Agnosia by University of London; M. Jane Riddoch North East London Polytechnic. Glyn W. Humphreys Birkbeck College in PDF and/or ePUB format, as well as other popular books in Psychology & History & Theory in Psychology. We have over one million books available in our catalogue for you to explore.
Information
1
Visual Object Recognition and its Disorders
We are surrounded by objects. Our lives are spent identifying, classifying, using and judging objects. Objects are tools, shelter, weapons; they are food; they are things precious, beautiful, boring, frightening, lovable . .. almost everything we know. We are so used to objects, to seeing them wherever we look, that is quite difficult to realise that they present any problem.
(Gregory, 1970; p. 11)
Introduction
The term âvisual object recognitionâ refers to our ability to apprehend the meaning of objects, our prior associations with them, and their uses, from vision. Normally, visual object recognition occurs at a glance, with such apparently effortless alacrity that it is indeed difficult to realise the complexity of the processes involved, or that understanding how we recognise objects should be such a problem. Most people feel that the visual world is simply given to them from the information relayed to the brain from the eyes, and that no more by way of explanation is needed. Yet such is the complexity of the processes involved that, even after more than a century of careful investigation, our knowledge of visual recognition and of how it is implemented in the brain remains all too incomplete. We are still some way from achieving the kind of detailed knowledge that would, for instance, allow us to build a machine to see and recognise objects in the manner that we do. What is certainly true is that the end product of visual recognition, our ability to act on and to negotiate the visual world appropriately, tells us little about the complex processes behind its production.
Various lines of investigation might be taken towards understanding how we recognise objects. For instance, one might attempt to build a machine capable of making the same responses as ourselves to visual input (such as identifying an object). In doing this, one would hope to glean some of the processes which might have to be involved in interpreting that input, irrespective of whether the interpretation is done by a human or a machine. Another approach might be to study the efficiency with which we recognise different types of objects under contrasting viewing conditions. For instance, one might present objects very briefly to normal observers and look at the kinds of misidentification errors they make. In doing this, one might hope to learn about the beginnings of the perceptual process (about which kind of information is picked up first, and so on). Thus when observers misidentify a drawing of a donkey as a dog, for example, one might conclude that they have picked up information about the general shape of the picture rather than its detailed features. It would then follow that general shape information is picked up âearly onâ in perceptual processing. A third approach is to study the selective losses of visual function which can occur after damage to the visual system, and in particular after damage to the brain. The hope is that, in such cases, we are given a privileged view of how the normal system operates in isolation from the damaged component(s). We may thus learn something about the nature of the various functions by examining how their loss affects recognition.
This book serves to illustrate this third approach. In it we document the case of a patient who, due to a stroke, suffered highly selective brain damage affecting only his visual recognition ability. The patient, John, is remarkable in many respects. From a purely academic point of view his case is of great interest because the effects of the brain damage have been so selective, disrupting only certain aspects of visual recognition. Johnâs case may therefore illuminate some of the processes in vision, and the ways in which these processes are organised in the brain. More generally, his case demonstrates just how devastating the selective loss of abilities such as visual object recognition can be, and yet how people adapt to cope with such losses. We hope the lessons to be learned will not be solely academic.
What is Visual Agnosia?
Before we can begin to understand the kinds of problems John encounters when trying to recognise objects, and why these problems might arise, we need to put his case in the context of previous studies of similar cases. This will give us a framework within which we can understand his recognition disorder.
Patients who, following brain damage, have problems in recognising formerly familiar objects from sight are said to suffer visual agnosia. Somebody with this disorder is able to âseeâ objects; that is, they may be able to describe the parts of an object or they may be able to copy a picture of it, but they have no idea what the object is or how it might be used. What makes the condition even more puzzling is that if the patient is allowed to hold and to feel the object, he or she is often able to identify it. Cases of visual agnosia are extremely rare, although they have been documented since the late nineteenth century. Even rarer still are detailed analyses of what such cases may tell us about how visual recognition normally works. One early account of visual agnosia is that of Charcot (1883). He described the case of a well-educated man, known for a remarkable visual memory, who, as a result of a stroke, suddenly lost the ability to recognise and visualise places, colours, forms, and human faces. He no longer recognised familiar aquaintances (not even his wife and children), and he no longer recognised his own face. Charcot describes an occasion when this patient was standing in the passage of a public place and found that his way was blocked. He was about to excuse himself to the man who stood in front of him when he realised it was only his own reflection in a mirror.
Around the same time, a physiologist, Hermann Munk, who was involved in the experimental study of the functions of the various parts of the brain, removed the posterior parts of the brain (the occipital lobes) in a dog and found that the dog subsequently behaved in a similar way to the patient described by Charcot (Munk, 1881). The dog was found to have no abnormality of hearing, smell, taste, movement or sensation. It moved about quite normally both indoors and in the garden and could apparently still âseeâ objects to the extent that it would walk around those placed in its way; it would neither walk into them nor trip over them. However, the dog no longer appeared to recognise the people that it had known well in the past and it would ignore other dogs. It no longer appeared to recognise its food and water bowls and would go around them as if they were obstacles that had been placed in its path (though it would respond to the food if near enough to smell it). The sight of the whip which, in the past, would drive the dog into the corner, no longer frightened it at all. The dog had also been trained to present a paw in response to a hand waved in front of its eyes; now, however much a hand was waved, the dog would ignore it. Unlike human subjects with visual-object agnosia, Munkâs dog did not suffer its disorder for long. Within a few weeks it could once again recognise its master and other dogs and would respond in a normal fashion to them.
Charcot and Munk accounted for the disorder which had occurred in both man and dog in an essentially similar way. Munk devised the term âmind-blindnessâ, by which he meant that there was a loss of visual ideas and visual memories, so there was no longer an ability to recognise what was seen. However, it was accepted that both man and dog could see because they could walk about and negotiate obstacles quite apropriately.
Visual Recognition and the Brain
Given the existence of patients with such a selective problem in visually recognising objects, a number of questions spring to mindâsuch as: How could damage to the brain generate such a problem? To answer such questions, we need to consider some points about the nature of brain function and organisation.
The brain is a complex structure, containing about 100,000,000,000 cells or neurons. It is divided into different sections, which have different evolutionary histories and which serve different purposes. For instance, the top part of the brain, termed the cerebrum, is thought to be concerned with our higher mental processes. The cerebrum sits above other structures which are, in evolutionary terms, rather older, such as the thalamus (a kind of sensory relay station) and the cerebellum (concerned with the coordination of voluntary movement, balance etc.). The cerebrum itself is divided down the middle into two cerebral hemispheres, with communication between the hemispheres made possible by a connecting structure termed the corpus callosum. The surface layer of neurons within each hemisphere is called the cerebral cortex, which folds over and under itself many times, rather like a very crumpled sheet. Enclosed beneath the cortex is the so-called âwhiteâ matter, consisting of masses of nerve fibres which relay messages within the brain and between the brain and the body. These structures are illustrated in Fig. 1.1.
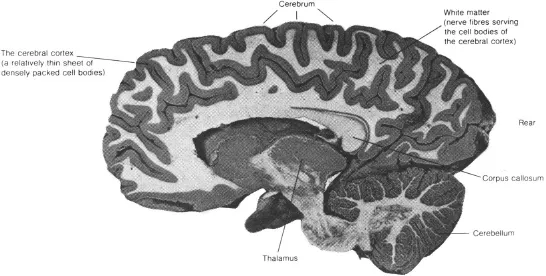
Fig. 1.1.The human brain, sliced from front to rear through the right cerebral hemisphere (from Frisby, 1979).
The way in which messages are relayed from the eyes to the brain is not as straightforward as one might initially think. Messages are passed from each eye along an optic nerve. The optic nerves join at the optic chiasm, where some fibres from each nerve cross over and connect to the cerebral hemisphere on the opposite side. By means of this partial crossing-over of nerve fibres, fibres to the left of the central part of both retinae project back to the left cerebral hemisphere, and fibres to the right of the centres of the retinae project to the right hemisphere. Now, due to the laws of optics, all stimuli to the right of the line of sight (or fixation) will fall on the left halves of both retinae, while stimuli to the left of fixation fall on the right halves of both retinae, as illustrated in Fig. 1.2. This means that stimuli to the left of fixation project information directly to the right cerebral hemisphere, whilst stimuli to the right of fixation project directly to the left hemisphere (by way of the lateral geniculate nucleus and the optic radiations; see Fig. 1.2). The optic radiations are the final structures involved in relaying messages to the cortex, and they project primarily to what is termed the striate cortex, or visual area 1 (V1), within the occipital lobe at the posterior end of the brain (see Fig. 1.3). Fibres from the striate cortex then project forward in the brain to adjacent regions in the prestriate cortex (so called because this area of cortex lies just in front of the striate cortex). In addition to this primary visual pathway, messages are relayed directly from both retinae to a mid-brain structure termed the superior colliculus (Fig. 1.2). The superior colliculus, unlike the striate cortex, does not appear to be directly involved in visual recognition; rather it appears to be involved in the way in which we attend to visual events, and particularly in the guidance of the eyes to novel stimuli.
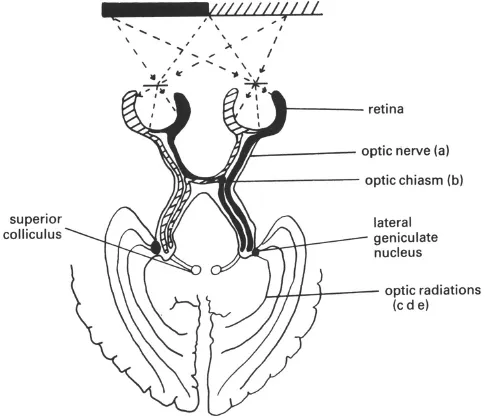
Fig. 1.2.The visual pathways to the brain. Damage to the different sites shown produces the following visual field defects (in all cases, lesions of the same sites on the left side of the brain will produce equivalent field defects, but in the right rather than the left visual field):
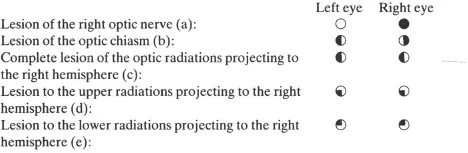
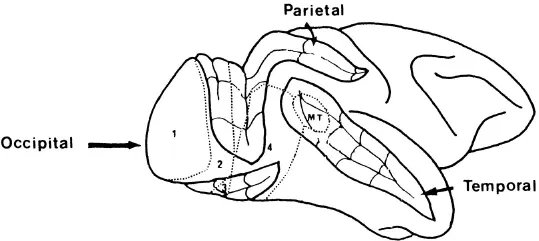
Fig. 1.3.Side-view of the right hemisphere of a rhesus monkey, showing the position of various visual areas. The front of the brain is on the right. Four of the major convolutions in the brain have been âopened outââto reveal their recesses. The numbers and initials refer to different identified visual areas (modified from Cowey, 1985). visual areas which are not mentioned in the text are not marked.
Damage to various parts of the visual pathway can produce characteristic âvisual field defectsâ, where regions of the visual field are rendered effectively blind to light (see Fig. 1.2). One might thus initially think that visual agnosia, the selective problem in recognising objects visually, is due to a field defect of this sort. However, this does not appear to be the case. These field defects do not usually cause recognition problems in their own right since vision remains intact in the remaining areas of the field. Providing that an object falls within those remaining areas, it will be recognised.
One of the most significant advances in understanding the way in which visual recognition is implemented in the brain came about in the early 1960s with the work of two physiologists, David Hubel and Torsten Wiesel. Hubel and Wiesel (e.g., 1962, 1968) conducted a series of studies in which they recorded the responseâs of single cells in the striate cortex of the cat to various stimuli projected to different parts of the retina. They found that the cells demonstrated distinct preferences for specific stimuli, being greatly excited by some stimuli and not at all by others. Of special interest was the finding that some cells responded strongly to the edges of stimuli and to light or dark bars at particular orientations. Also, different cells preferred edges in different orientations and the responses of these cells waxed and waned depending on how close the orientation of the actual edge was to the orientation preferred by the cell. Further, each cell was associated with a particular part of the retina, so that information concerning the location of each edge in space was preserved. By preserving location information, the cells in the striate cortex may be said to contain a âmapâ of the retina. These cells have commonly been labelled âfeature detectorsâ, since they were initially thought to be capable, individually, of signalling the presence of a particular kind of edge in any visual stimulus. It turns out that such a view is almost certainly wrong, for reasons we discuss in more detail in Chapter 3. Nevertheless, the discovery of these cells did provide some inkling of the initial coding processes upon which visual recognition is based, indicating that recognition initially involves coding the edges and contours of objects. However, recognition clearly cannot be based on this coding alone, since we cannot recognise objects from single edges. Recognition must involve considerable elaboration of the edge and bar information coded in the primary visual cortex, area V1.
Since Hubel and Wieselâs pioneering work, a good deal of research has been devoted to generating a picture of the stimulus preferences of the cells in the cortex concerned with visual processing. The evidence points clearly to the involvement of many brain areas in the processing of a given object. For instance, researchers have documented eight different cortical areas concerned with vision in the owl monkey (e.g., Weller & Kaas, 1985), eleven in the macaque monkey (e.g., Van Essen, 1985) and thirteen in the cat (e.g., Tusa, Palmer & Rosenquist, 1975); and we may presume that the human visual system is at least as complex as that of the monkey or the cat. These different visual areas extend from the striate cortex (V1) into the pre-striate cortex in the occipital lobe, and also further forward into the parietal lobes (at the top of the brain) and temporal lobes (at the side of the brain; see Fig. 1.3). The areas can be identified because they contain, amongst other things, differing cell types and complete or partial replications of the map of the retina (i.e., some seem to mirror the organisation of the cells in the retina, whereas others are only related to some of the cells of the retina). We might then ask what the need is for this multitude of visual areas, many of which contain cells tuned to the same area of the retina as cells in other visual areas. One likely answer is that these different areas are the sites of increasingly refined processing of visual images. Further, there are different connections from area V1, the source of the major projection from the retina, to a number of other visual areas (see, for example, the work of Zeki, 1978). These different connections allow the possibility that the further processing of images is conducted simultaneously in the various visual areas, with each area doing a different job. The main sets of connections from V1 go either to the parietal or to the temporal lobe, and it has been suggested that these two secondary pathways serve different purposes (see Ungerleider, 1985): the parietal pathway being concerned with coding the location, movement and spatial relations between stimuli, the temporal pathway with the actual recognition of the stimuli. Support for this suggestion, and also for the further differentiation of areas within the parietal and temporal pathways, comes from more recent studies using single-cell recording techniques. For instance, the temporal pathway contains, along with other areas, two areas termed V2 and V4 (Fig. 1.3). Some cells in V2 respond specifically to differences in the positions of stimuli in the images from the left and right eyes (Fischer & Poggio, 1979). These differences, termed âbinocular disparitiesâ, are one of the main cues we use to help us judge whether objects are at different distances from us (for more detail on this, see Chapter 4). Cells in V4, on the other hand, r...
Table of contents
- Cover
- Title Page
- Copyright
- Contents
- Preface
- 1. Visual Object Recognition and its Disorders
- 2. The History of a Case of Visual Agnosia
- 3. The Process of Visual Object Recognition
- 4. Some Experimental Investigations
- 5. Other Visual Problems in Agnosic Patients
- 6. Some Conclusions About Agnosia and Visual Recognition
- 7. Living with Agnosia
- References
- Author Index
- Subject Index