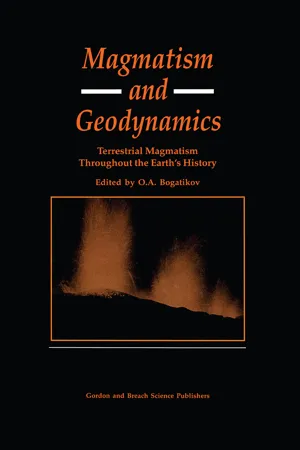
eBook - ePub
Magmatism and Geodynamics
Terrestrail Magmatism Throughout the Earth's History
This is a test
- 512 pages
- English
- ePUB (mobile friendly)
- Available on iOS & Android
eBook - ePub
Book details
Book preview
Table of contents
Citations
About This Book
Magnatism is the only true endogenic process for generating new material on the Earth's surface. Obviously, magmatism and tectonic movements are reflections of geodynamics, that is, physical processes which occur in deep-seated environments. What are the interrelationships between magmatism and tectronics? How did the character of terrestrial magmatism change through time and are there any irregularities in this process?
Frequently asked questions
At the moment all of our mobile-responsive ePub books are available to download via the app. Most of our PDFs are also available to download and we're working on making the final remaining ones downloadable now. Learn more here.
Both plans give you full access to the library and all of Perlego’s features. The only differences are the price and subscription period: With the annual plan you’ll save around 30% compared to 12 months on the monthly plan.
We are an online textbook subscription service, where you can get access to an entire online library for less than the price of a single book per month. With over 1 million books across 1000+ topics, we’ve got you covered! Learn more here.
Look out for the read-aloud symbol on your next book to see if you can listen to it. The read-aloud tool reads text aloud for you, highlighting the text as it is being read. You can pause it, speed it up and slow it down. Learn more here.
Yes, you can access Magmatism and Geodynamics by O A Bagatikov in PDF and/or ePUB format, as well as other popular books in Physical Sciences & Mechanics. We have over one million books available in our catalogue for you to explore.
Information
CHAPTER 1
PRINCIPLES OF PETROLOGICAL ANALYSIS OF IGNEOUS ROCKS
Igneous processes are responsible for heat and mass exchange in the outer layers of the terrestrial-group planets. Igneous melts originating at depth bring magma to the surface to form planetary crust. The constant loss of low-melting-point components from the deeper layers leads to differentiation of the planets’ bodies. Thus it can be predicted that the igneous melts changed continuously over time, and this can provide important information about the nature and rate of planetary evolution. It is also evident that the melt composition will differ under certain geodynamic conditions, depending on the distribution of ascending and descending convection cells, whether or not differentiation has already occurred, and the melt’s involvement in later reworking. This explains the close relationship between certain types of igneous activity and tectonic settings.
Certainly, magmatism is a complex phenomenon, and therefore the interpretation of particular types of igneous activity and their significance in the context of planetary evolution (in this case that of the Earth) requires the application of geophysical, petrological and isotope-geochemical methods.
Undoubtedly, the history of terrestrial magmatic processes should be analysed from the standpoint of both intrusive and volcanic rocks. It is therefore necessary to discuss briefly the interrelated issues relevant to the paragenesis of igneous rocks, and how the intrusive equivalents of volcanic rocks can be recognized.
1.1 THE IMPORTANCE OF SOLIDIFICATION PROCESSES IN THE PETROGENESIS OF IGNEOUS ROCKS
1.1.1 The Differences Between Volcanic and Intrusive Rocks
Lavas and intrusions are a result of crystallization of the same magmas. However, their emplacement takes place under quite different conditions, leading to different textures and structures. Volcanic rocks are generally poorly crystallized; differentiation does not usually occur except for rare cases of superheated Early Precambrian komatiite or picrite melts. Intrusive igneous rocks are generated under quite different conditions, especially within large intrusive chambers, accompanied by intense fractional crystallization.
Layered intrusions are ideal for studying the solidification and differentiation processes, because their original structures and textures are easily detected in the field. As has been discussed repeatedly (Jackson, 1961; Wager and Brown, 1968; Sharkov, 1980; etc.) the solidification of large intrusions takes place during the upward movement of a thin (only metres thick) crystallization zone. This process owes its character to the fact that the adiabatic PT gradient in silicate melts is steeper than that of the melting point related to the barometric pressure (Jeffries, 1929; Jackson, 1961). Therefore, at any specific moment in time, crystallization within the magma chamber occurs only at its base, because most of the melt is superheated with respect of liquidus (Fig. 1.1). This relationship is of paramount importance for the solidification of large volumes of magmatic melt. According to Jeffries (1929) it must have controlled the solidification of the molten planets during the formation of the Solar system.

However, the picture is different in the marginal parts of intrusions. There, solidification proceeds from the margins to the core due to the marked thermal gradient at the contact with the cold country rocks (Fig. 1.2). This pattern of emplacement affects the overall structure, i.e. conformable with the contacts of the marginal series with the country rocks and autonomous with respect to the contacts of the major central series (Fig. 1.3), whose formation mainly controls the geochemical evolution of melts in the body of the intrusion (Wager and Brown, 1968; Sharkov, 1980).

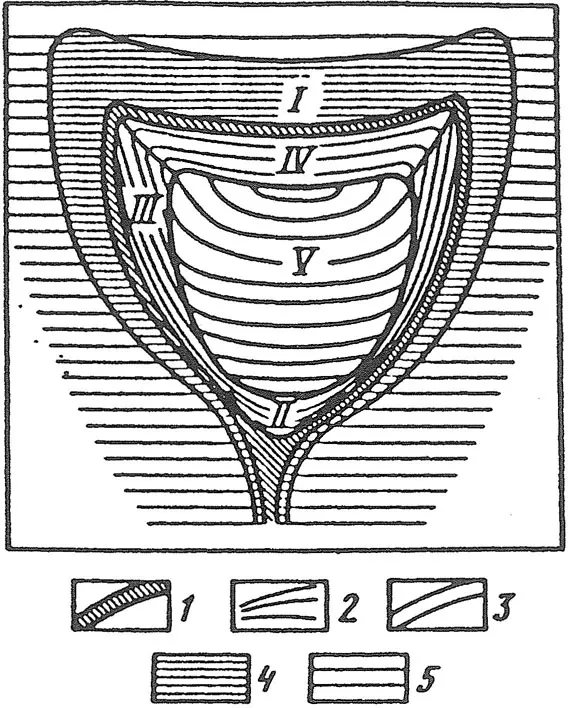
The upper edge of the crystallization zone (Fig. 1.4) at the base of the magma chamber corresponds with the liquidus of the main melt volume, i.e. the liquidus solid phases precipitate here. They form about 70–75% of the crystallization zone with residual liquid among them. The lower edge of the zone (the crystallization termination front) corresponds with the solidus isotherm of residual melt in it. Hence, two types of crystals occur in intrusive rocks (cumulates). The euhedral crystals of the liquidus phases are known as cumulus minerals and the anhedral solidus minerals between them are called intercumulus minerals (Wager and Brown, 1968). The crystallization zone moves continuously upward when most of the melt is being cooled and crystals which settled out earlier are covered by later accumulations. The constant loss of high-temperature phases changes the composition of the melt and leads to the appearance of new, lower-temperature mineral assemblages as the liquidus phases, which are responsible for the occurrence of primary layering.
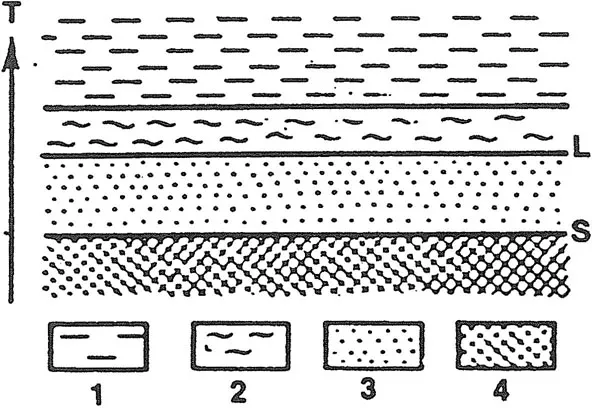
Another consequence of this directed solidification is a rhythmic layering represented by the regular alternation of different cumulates, gabbro and pyroxenites, for example. Most investigators suggest that they derived as a result of gravity differentiation. However, a special granulometric study of rhythmic layered rocks has shown that their cumulus phases are not equivalent in their hydraulic properties and could not have such an origin (Jackson, 1961; Sharkov, 1980). In fact, they are defined by alternation of high- and low-temperature cumulates — a type of temperature-concentration waves, characteristic of a dissipative system (Glensdorf and Prigozhin, 1973), which consolidated intrusions are. These waves are formed by the removal of heat from the system which occurs faster than the assimilation of the residual liquid ejected from the crystallization zone, by the main volume of the melt. As a result the liquid is concentrated ahead of the solidification front in the form of a diffusion zone (Fig. 1.4), leading to the concentration supercooling of the front of the beginning of crystallization, to its instability of the melt and the formation of a rhythmic pattern (Sharkov, 1980).
In lavas, in contrast to intrusions, the liquidus phases form phenocrysts, subliquidus phases form microphenocrysts and the residual melt forms a ground-mass recrystallized to differing degrees, all of which can be seen in a single thin section. In lavas, these phases correspond with different stages of crystallization, whereas in intrusions they form separate layers of rocks similar in structure and texture and tend to be aligned upward. Naturally, each cumulate layer differs in composition from that of the melt because the layers are formed by either liquidus or subliquidus phases, and so on, up to the very low-temperature assemblages, i.e. the original melt has been fractionated.
Therefore, despite the close genetic relationship between intrusive and volcanic rocks they differ greatly in both their textures and structures. Moreover, unlike the solidification of lavas, the crystallization of intrusions may occur at different depths, which influences the way in which mineral assemblages segregate, even for the same composition of initial melts. For these reasons, the reconstruction of the intrusive equivalents of volcanic rocks poses a particular challenge.
In terms of magmatic series, the study of intrusions, especially layered types, provides important additional information about the true extent of a series, because all the rock types occur in the single cross-section. Therefore, in intrusive series the differentiation process can be observed as a whole, unlike in volcanic series, where each lava flow is an individual body, and so the question arises as to whether or not the associated rocks derived from the same magmatic source.
Until recently, intrusives were considered to be closed systems, i.e. they were believed to result from a single intrusion of magma into the chamber. However, detailed geological and isotopic data from large layered intrusions (Bushveld, Stillwater and Monchetundra, etc.; see Chapter 5) suggest that they represented open systems during the crystallization process and that they resulted from multiple influxes of new batches of the melt into the magma chamber of solidified intrusions. Therefore, the composition of magma in these chambers can change not only due to fractional crystallization, but also due to the replenishment of new batches of fresh melt. Obviously, the fresh melt could be derived from both the magma generation zone and the intermediate crustal chambers.
Apparently, intermediate chambers were represented by large, solidifying intrusions. As a result of convection, heat is mainly lost through the top of the magma chamber. Therefore the country rocks at the top of the chamber could be melted by high-temperature primary melts; a newly formed melt can become involved in convection and become mixed in with the main volume of melt in the magma chamber. Thus, magma systems display very complex structures, and hence the origin of a magmatic series may not be as simple as has been thought previously.
Based on available geological and geophysical data, it is thought that lava is mainly ejected from solidified intermediate magma chambers, i.e. it has already been segregated from the main volume of the melt. This melt could well be in equilibrium with segregating solid phases (cumulates), whose fragments often occur as cognate xenoliths. Therefore, lava phenocrysts could reflect the composition of cumulates at the time of separation of a given batch from the main melt. Hence, intrusives can provide important information on the specific mechanism responsible for the formation of volcanic assemblages, the dynamics and features of development of both magma chambers and magma generation zones and, in some cases, even about the mechanism of melt formation of the whole magmatic series. Some...
Table of contents
- Cover
- Half Title
- Title Page
- Copyright Page
- Table of Contents
- List of Contributors
- Acknowledgements
- Introduction
- Abbreviations
- 1 Principles of petrological analysis of igneous rocks
- 2 Magmatism in the context of the present-day tectonic settings
- 3 Magmatism of Phanerozoic fold belts
- 4 Phanerozoic anorogenic magmatism
- 5 Early Proterozoic magmatism and geodynamics — evidence of a fundamental change in the Earth’s evolution
- 6 Magmatism and geodynamics in the Archaean E.V. Sharkov and O.A. Bogatikov
- 7 General features of magmatic evolution throughout the Earth’s history
- 8 Terrestrial and lunar magmatism: An evolutionary overview
- 9 The sources and origin of magmas
- Conclusions
- References
- Index