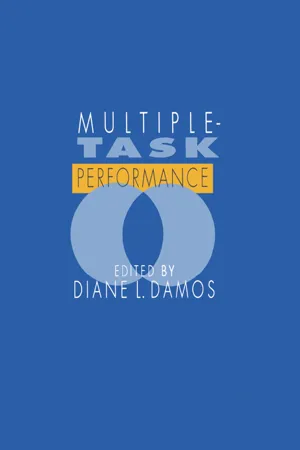
This is a test
- 469 pages
- English
- ePUB (mobile friendly)
- Available on iOS & Android
eBook - ePub
Multiple Task Performance
Book details
Book preview
Table of contents
Citations
About This Book
This book deals with theories of multiple-task performance and focuses on learning and performance. It is primarily for professionals in human factors, psychology, or engineering who are interested in multiple-task performance but have no formal training in the area.
Frequently asked questions
At the moment all of our mobile-responsive ePub books are available to download via the app. Most of our PDFs are also available to download and we're working on making the final remaining ones downloadable now. Learn more here.
Both plans give you full access to the library and all of Perlego’s features. The only differences are the price and subscription period: With the annual plan you’ll save around 30% compared to 12 months on the monthly plan.
We are an online textbook subscription service, where you can get access to an entire online library for less than the price of a single book per month. With over 1 million books across 1000+ topics, we’ve got you covered! Learn more here.
Look out for the read-aloud symbol on your next book to see if you can listen to it. The read-aloud tool reads text aloud for you, highlighting the text as it is being read. You can pause it, speed it up and slow it down. Learn more here.
Yes, you can access Multiple Task Performance by D Damos in PDF and/or ePUB format, as well as other popular books in Tecnología e ingeniería & Ingeniería industrial. We have over one million books available in our catalogue for you to explore.
Information
PART I
Models and methodology
Introduction
The primary purpose of this section is to give the reader a broad perspective on the types of models that currently are used to describe multiple-task performance. A secondary purpose is to introduce some of the most common methodological problems encountered in assessing multiple-task performance.
The multiple resource model is presented first. Christopher D. Wickens begins with a discussion of the concept of processing resources and the performance resource function. He then briefly describes some of the earlier performance models, expanding the concept of processing resources to dual-task situations. The remainder of the chapter describes the multiple resource model in detail and presents supporting evidence.
Krishna R. Pattipati and David L. Kleinman present engineering models that describe performance in multiple-task supervisory control situations. The authors acquaint the reader with the skills required in multi-task supervisory control situations. Next, they review display monitoring and multi-task decision-making models. Pattipati and Kleinman conclude by describing how multiple-task supervisory models could be useful analysis tools in man-machine system design.
Mark Detweiler and Walter Schneider present a very different type of dual-task performance model. The model described in this chapter is a connectionist/control model, which is related to parallel distributed processing models. The most striking characteristic of the model presented here is its use of neurally feasible architecture. Detweiler and Schneider describe connectionist/control architecture, then cover their model in detail, including the phases the model goes through as it learns single and dual tasks. The authors conclude with a discussion of the skills and strategies that can be learned under dual-, but not single-, task conditions.
Diane L. Damos focuses on common dual-task methodological problems. One probem – distinguishing a dual-task combination from a complex single task – is discussed first. Next, Damos focuses on several aspects of the second common methodological problem, practice. These include identifying stable levels of performance and using efficient training schedules to reach stable dual-task performance. The third methodological problem addressed by Damos concerns presenting concurrent feedback to control task priorities.
Chapter 1
Processing resources and attention
Christopher D. Wickens
How do we timeshare, and how do we cope when we must perform two or more activities in a short period of time? There are probably several mechanisms that determine our successes and failures in this endeavor (Wickens, 1989). First, it is clear that good scheduling of our time, and efficient switching between activities are important ingredients of success. If given 10 minutes to perform two 5-minute tasks, the operator will achieve success if she makes full use of the available time (efficient scheduling) and wastes no time in switching from one activity to the next. On the other hand, if given only 7 minutes to complete the two 5-minute tasks, such a schedule will probably force the person to engage in concurrent processing. If this is the case, then there are three further factors that will influence the effectiveness of multiple-task performance: confusion of task elements, cooperation between task processes, and competition for task resources.
Confusion results when elements for one task become confused with processing of another task because of their similarity. Thus, if I am trying to listen to two speakers at once, whose voices are of similar quality, and who are discussing a similar topic, it is quite likely that I will mistakenly attribute the words of one to the message of the other (Navon, 1984). These confusions will diminish if the speakers differ more in the physical and semantic characteristics of their message.
Sometimes, high similarity of processing routines can yield a cooperation or even an integration of the two task elements into one. Thus, for example, when the pianist or drummer must implement two separate response streams, this task is made easier if they share a common rhythm (Klapp, 1979). Similarly, control of a horizontal and vertical axis in a tracking task is made easier if these are displayed by (and controlled with) one two-dimensional element (like tracking a fly with a fly swatter), than if two independent axes of control are employed (Fracker and Wickens, 1989).
Switching (Pattipati and Kleinman, chapter 2, this volume; Moray, 1986), confusion (Hirst and Kalmar, 1987; Navon, 1984; Navon and Miller, 1987), and cooperation (Duncan, 1979; Fracker and Wickens, 1989) are all important components of multiple-task performance and each will be discussed in more detail at the end of the chapter. The current chapter, however, focuses on a fourth mechanism – resources – which plays an equally critical role in accounting for task interference and also, as we shall see, has implications as well for the performance of a single task in isolation.
The resource concept in single-task performance
The resource concept is founded on the underlying assumption that the human operator has a limited capacity for processing resources that may be allocated to task performance. Two tasks demand more resources than one; therefore, timesharing can lead to a situation in which one or both have fewer resources than required, and hence, performance on one or both may deteriorate. In fact, the resources concept may be thought of as a ‘syndrome’ of phenomena that have a number of different manifestations, some intuitive, some empirical, and some based on plausible models. The concept gains validity from the convergence of these multiple phenomena. As I argue in this chapter, while each phenomenon, in isolation, may be explained by a different concept, all, together are best accounted for by a resource concept.
Intuitively the concept of ‘trying harder’ is familiar to all of us. As we try harder at a task, we invest more mental effort into its performance, and performance will often, though not invariably, improve. In this sense, resources are the mental effort that is invested to improve performance. Reinforcing this intuitive concept are the results of some experiments in which performance on tasks has improved either with instructions to try harder (Vidulich and Wickens, 1984), with more stringent performance criteria (Yeh and Wickens, 1988), or with motivational incentives offered in domains as diverse as signal detection (Watson and Clopton, 1969), manual control (Vidulich and Wickens, 1986), or test-taking performance (Johnson et al., 1984). The hypothetical function that underlies this relation between effort (resources invested) and performance, is known as a performance-resources function (PRF) (Figure 1.1) which will be the focus of later discussion.
While investing more effort will improve performance on a task of fixed difficulty, investing more effort will be necessary to maintain a constant level of performance on a task of increasing difficulty. Hence, it is more difficult to retain seven chunks of information in working memory than five, even though recall performance might be perfect in both cases. Correspondingly, our grammar and sentence structure may be equally impeccable when speaking a primary or secondary language, but the level of concentration necessary to sustain the latter will be a good deal higher (Dornic, 1980). In both of these cases, we may speak of a family of PRFs, as shown in Figure 1.1. All tasks may generate the same level of performance with full effort allocated to the task (resources investment), but easier tasks require fewer resources to achieve maximum performance.
The concept of effort, and its relation to performance and task difficulty, gains some empirical plausibility because of changes in physiological measures of arousal, and in subjective measures of effort, that correspond with circumstances in which the PRF model would predict their change (Kahneman, 1973). For example, measures of pupil diameter are found to correlate well with the demand on working memory imposed by mental calculations (Beatty, 1982) or by reaction-time tasks (Richter et al., 1983). Numerous investigators have found that changes in heart-rate parameters correlate with increases in task load (e.g., Derrick, 1988; Mulder and Mulder, 1981; Vicente et al., 1987). A convergence of resources and physiological measures with subjective measures is provided by findings that the same manipulations which give rise to physiological changes will typically create subjective assessments of higher effort (Yeh and Wickens, 1988). In addition to changing the difficulty of a task (which amounts to shifting between two or more PRFs in Figure 1.1), investigators have also measured subjective load as performance varies along a single PRF, by inducing various levels of effort investment through motivational incentives (Vidulich and Wickens, 1986; Yeh and Wickens, 1988). These studies have found that subjective ratings of effort closely correlate with instructions to try harder, and with the concomitant improvements in performance.
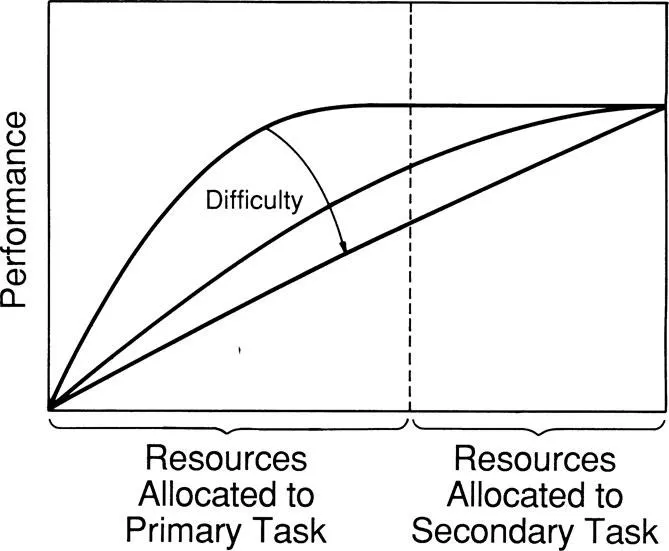
(From Norman and Bobrow, 1975).
These data, then, are all consistent with a rough ‘model’, describing the relation between performance (P), objective task difficulty (D), and resources (R) as having the form:
which is referred to as the ‘PDR’ model.
The model is not truly predictive, in the sense that absolute constants can be placed on either side of the equation, but is descriptive of the general relation, in the sense that holding any one term constant will describe the appropriate form of covariation between the other two. For example, as resources investment is held constant, increasing difficulty will decrease performance. The concept of effort or resources investment is of critical importance here, because it accounts for major aspects of either performance, or experience and physiological state measures, in a way that is consistent with the single model. Finally, it is important to note that the PDR relation above has been conceptualized entirely in the single-task domain, and has been related here directly to the physiology of arousal systems. That is, the basic validity of the resource concept is not derived from its ability to predict dual-task interference. However, the extent to which it does accomplish this prediction will serve to further enhance this validation process.
Later in this chapter I will address the dual-task domain, and ask whether the same variables that underlie the resource concept in single-task performance (investment, difficulty) are successful in predicting dual-task performance. However, before leaving the domain of single-task performance, it is worth highlighting some of the ways in which the resources concept as related to ‘mental effort’ has been used to explain other aspects of attention and cognitive p...
Table of contents
- Cover
- Half Title
- Title Page
- Copyright Page
- Table of Contents
- Preface
- List of contributors
- Part I Models and methodology
- Part II Learning and performance
- Part III Mental workload
- Part IV Individual differences
- Glossary
- Index