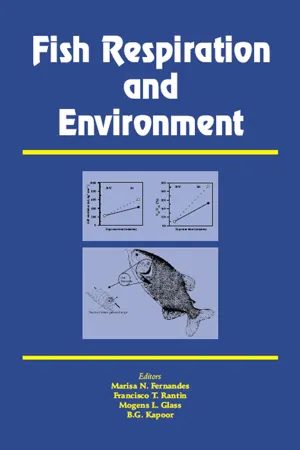
This is a test
- 408 pages
- English
- ePUB (mobile friendly)
- Available on iOS & Android
eBook - ePub
Fish Respiration and Environment
Book details
Book preview
Table of contents
Citations
About This Book
Gills of healthy fishes are their lifeline to meet the challenges arising from their changing environment: oxygen gradient, alkalinity, temperature fluctuations and the added pollutants. The diverse and ever changing aquatic environment has a major impact on the organization of various organ-systems of fishes. This book contains seventeen chapters
Frequently asked questions
At the moment all of our mobile-responsive ePub books are available to download via the app. Most of our PDFs are also available to download and we're working on making the final remaining ones downloadable now. Learn more here.
Both plans give you full access to the library and all of Perlegoâs features. The only differences are the price and subscription period: With the annual plan youâll save around 30% compared to 12 months on the monthly plan.
We are an online textbook subscription service, where you can get access to an entire online library for less than the price of a single book per month. With over 1 million books across 1000+ topics, weâve got you covered! Learn more here.
Look out for the read-aloud symbol on your next book to see if you can listen to it. The read-aloud tool reads text aloud for you, highlighting the text as it is being read. You can pause it, speed it up and slow it down. Learn more here.
Yes, you can access Fish Respiration and Environment by Marisa N Fernandes in PDF and/or ePUB format, as well as other popular books in Biological Sciences & Marine Biology. We have over one million books available in our catalogue for you to explore.
Information
CHAPTER
1
Adaptation of Gas Exchange Systems in Fish Living in Different Environments
S.M. Kisia* and D.W. Onyango
Authorsâ address: Department of Veterinary Anatomy, University of Nairobi, P.O. Box 30197, Nairobi, Kenya.
*Corresponding author: E-mail: [email protected]
INTRODUCTION
The survival of fish depends largely on its morphological, physiological and biochemical adaptations to the environment. One important system to the fishâs survival is that of gas exchange that should be able to efficiently obtain oxygen from the external environment in order to meet the metabolic needs of fish and eliminate gaseous wastes, chiefly carbon dioxide. The evolution of different structures used in respiration by fish has been taking place for the hundreds of millions of years that fish have been in existence and is likely to continue with changing environmental conditions and metabolic demands of fish. The evolution of diverse respiratory systems has enabled fish survive and reproduce in most of the aquatic habitats on earth. The aquatic environment normally contains a lower oxygen content than the terrestrial environment. Warmer waters have even less oxygen tension in comparison to cold waters. This is a constraint faced by tropical water fish when compared to those of temperate waters. Hypoxia and anoxia have been common constraints during the evolutionary history of tropical water fishes. Similarly, stagnant waters compared to moving and stirred water will contain less oxygen. The presence of organic matter or pollutants in water can further reduce the quantity of oxygen, thereby creating oxygen-poor zones as bacteria that oxidize the organic matter, in turn, reduce the available oxygen due to their respiratory activity.
To face the challenges of an aquatic environment, fish have to migrate to suitable environments, adjust their metabolic requirements, evolve a system that will enable them to get enough oxygen so as to meet metabolic needs or become endangered. For example, a genus of the South American electric eels, Electrophorus has developed a network of blood vessels in its mouth as a result of low concentrations of oxygen in the water they occupy in the Amazon basin. These fish periodically rise to the surface to gulp air into their mouths. The African lungfish (Protopterus) has to occasionally gulp air for survival and, during dry periods, it has to cocoon in the mud of dried up river beds, breathing through a small opening that leads to the surface.
Based on the diverse environments they live in, fish have evolved various respiratory structures they use mainly or in combination with others to meet their oxygen requirements. The purpose of this chapter is to examine the various gas exchange structures that have evolved in fish including gills, lungs, swim (gas) bladders and other accessory air-breathing organs and relate their use as respiratory structures to the different environments occupied by fish.
Gills
Gills are the primary organs of respiration in fish. Apart from performing the chief function of gaseous exchange, gills play important roles in osmoregulation and excretion of nitrogenous metabolic wastes. Although external gills are found in the larvae of many fish such as the torpedo (Torpedo marmorata) and the spiny dogfish (Squalus acanthians), internal gills are found in most fishes. Internal gills have undergone various structural and functional modifications depending on environmental conditions, metabolic activity and dependence of fish on these structures for respiration.
The living agnathans (jawless fish) such as lampreys and hagfishes that suck blood from living fishes or eat the flesh of dead or dying fishes have evolved a pharynx which is divided longitudinally into a dorsal food passage (esophagus) and a ventral blind ending respiratory tube. Internal gill slits arise from the ventral tube so that when the fish are feeding, water is continuously pumped in and out of the branchial pouches. Oxygen then diffuses into the filaments (primary gill lamellae) that line the branchial pouches.
The evolution of a countercurrent system of gas exchange whereby blood in the gill secondary lamellae and water flow in opposite directions enables the fish to obtain the maximal possible amount of oxygen from the water. The amount of oxygen that diffuses into the gills can be as high as 80% of the oxygen dissolved in water ventilating the gills as compared to about 25% of oxygen utilized by mammals from inspired air. Fish spend a lot of energy in ventilating the gills with water and this can account for up to 10 to 25% of their oxygen uptake, when compared to 1â2% spent by man in ventilating the lungs (Hughes, 1965). During ram ventilation in fast-swimming fish such as lamnid sharks and tunas, forward motion (when their mouths are open) is used to enhance water flow across the gill filaments. Most fast-swimming sharks have lost or reduced spiracles, whereas bottom-dwelling skates and rays have enlarged spiracles as most of the water that ventilates the gills enters the pharynx through these structures.
Gill Surface Area and Oxygen Diffusion
The surface area and oxygen diffusion distances of the gill secondary lamellae (gas exchange sites) vary in different fish, depending on their metabolic needs. Active pelagic tuna and menhaden have secondary lamellar surface areas of 3151 mm2 (Muir and Hughes, 1969) and 1241 mm2 (Hughes, 1984), respectively, as compared to 18 mm2 in the much less active coelacanth (Latimeria chalumnae) (Hughes, 1980), 117 mm2 in the torpedo (Hughes, 1978) and 151 mm2 in the sluggish, bottom-dwelling toadfish (Hughes, 1984). Active fish such as the yellowfin tunny and mackerel have short diffusion distances over their gill respiratory surfac area of 0.17â1.13 Îź and 0.60â3.63 Îź, respectively (Hughes, 1970). This distance has been estimated at 5â6 Îź in the coelacanth (Hughes, 1972).
Since water and salts are lost or gained through the gills depending on the concentration of solutes in the water and body fluids, fish are faced with the problem of ventilating or perfusing their gills to a level that is only necessary to meet their oxygen metabolic requirements. Such a mechanism is applied in fish such as the Lake Magadi tilapia Alcolapia grahami (Maina et al., 1996) at the peak of daytime when water is supersaturated with oxygen as compared to almost anoxia later in the night (Narahara et al., 1996). The process of preferential perfusion of gillsâhence, partial participation in diffusionâhas been noted in other fish (Randall et al., 1972; Booth, 1978; Nilsson, 1986). The surface area of secondary lamellae that is ventilated can also be adjusted by contraction and relaxation of adductor and abductor muscles of the gills (Bijtel, 1949) as well as vascular shunts that divert some blood directly from affarent to efferent branchial arteries. Such blood bypasses the secondary lamellae. When the adductor muscles of a gill contract, water flows between the filaments of adjacent gills thus avoiding the secondary lamellae that are the respiratory gas exchange surface areas. The gill surface area that is exposed to ionic and water gain or loss is thus reduced. Contraction of the abductor and relaxation of the adductor muscles exposes a greater gill surface area to water.
ACCESSORY RESPIRATORY ORGANS
Accessory respiratory organs have enabled bony fishes belonging to more than 50 genera access oxygen directly from the air. These structures could have evolved as a result of the inability of gills to meet the gaseous matabolic demands of fish in water with low oxygen tension such as warm pools, swamps with a lot of decaying matter or stagnant water. Several changes have occurred with transition from aquatic to aerial respiration, including morphological and physiological changes in gas exchange, regulation of ions, excretion of nitrogenous wastes and acid-base balance. An obligate air-breathing fish such as Arapaima gigas of river Amazon that undergoes transition from water to air breathing during development and the accompanying changes in gill development offers a good model system in understanding the water to air transition in respiration (Brauner et al., 2004).
Lungs
The three surving genera of the order Dipnoi and freshwater bichirs Polypterus (primitive chondrosteans) have developed a pair of long hollow sacs (lungs) in the dorsal part of the abdominal cavity. These lungs originate as ventral evaginations from the floor of the anterior part of the digestive system. The dipnoan lungfishes include Neoceratodus of Australia, Protopterus of Africa and Lepidosiren of South America and live in tropical rivers and ponds. The lungs are thought to have evolved in the Devonian when bodies of water become stagnant and hypoxic and would periodically dry up; hence, fish needed an accessory respiratory organ to supplement the gills in any gaseous exchange. Protopterus and Lepidosiren can also survive the dry season, during which they undergo aestivation by forming cocoons that are surrounded by mucus, leaving small tubes that lead to the surface of a dried up river bed or pond for breathing. The fish also lower metabolic rates and excrete urea instead of the more toxic ammonia at such times (Mommsen and Walsh, 1989).
Neoceratodus is a facultative air breather that uses its lungs to supplement the gills when the water becomes hypoxic. During hypoxic conditions, the fish shows an increase in gill-breathing frequency, that is followed by an increase in the number of air breaths together with increased pulmonary blood flow (Fritsche et al., 1993) as well as increased haemoglobin oxygen affinity (Kind et al., 2002). Protopterus and Lepidosiren are obligatory air-breathers due to the thickness of their gill epithelia and reduced gill respiratory surface areas (Laurent, 1996). These fish rely on their lungs for about 90% of their oxygen supply (Johansen, 1970). The internal lung septae are more elaborate in Protopterus which has lost its first two gills. The frequency of lung ventilation in lungfishes is low, leading to accumulation of carbon dioxide which is eliminated by gills, since it is extremely soluble in water.
The presence of both branchial and pulmonary circulatory systems in lungfishes differs from that of fish that entirely rely on their gills for respiration and represents a transition from which the tetrapod mode of respiration has evolved. The ductus arteriosus, pulmonary artery vasomotor segments and gill shunts (Fig. 1.1) are useful structures in the preferential perfusion of the lungs or gills in dipnoan fish (Laurent et al., 1978). The ductus arteriosus connects anterior branches of the dorsal aorta with efferent branchial arteries that eventually give rise to pulmonary arteries. Pulmonary artery vasomotor segments are a thickening of the middle layer in the artery starting from the ductus arteriosus caudally over one-third of the artery. Gill shunts are large arteries of arches II and III (lack gills) with thick walls and connect the afferent and efferent arteries. Gill shunts greatly reduce the amount of blood that flows into the gill filaments and the secondary lammellae. During air and aquatic respiration, the pattern of circulation in the lungfish alternates between that of a tetrapod and a fish (Fishman et al., 1985). Figure 1.1 shows how the two systems operate when lungfish use aquatic and aerial respiration. When a lungfish relies on its gills for gaseus exchange exclusively, the pulmonary artery vasomotor segments (P) and the gill shunts (GS) are closed. The ductus arteriosus (DA) opens in the process. Blood is able to flow through the gill filaments and the efferent branchial arteries to the dorsal aorta. The reduction in pulmonary blood flow will result in reduced pulmonary venous return to the heart, which is compensated for by a right-to-left intracardiac shunt-through the incomplete partitioning of the ventricle. During pulmonary respiration, the pulmonary artery vasomotor segments and gill shunts are open, whereas the ductus arteriosus is closed. There is decreased flow of blood to the gill filaments and an increase in flow to the lungs. The right-to-left intracardiac shunt is reduced in the process. Burggren and Johansen (1986) have reviewed the cardiorespiratory morphology and physiology during aerial and aquatic respiration particularly those features that distinguish Neoceratodus from both Protopterus and Lepidosiren and lungfishes in general from aquatic vertebrates.
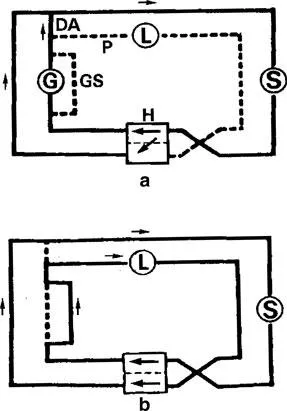
Swim (Air) Bladder
The swim (air) bladder in most fish is thought to have evolved from the lungs of ancestral bony fishes. Such fish have lived in oxygen-rich waters and conversion of the swimbladder to a hydrostatic organ was favored. Also known as the air bladder or ballast organ, this organ also plays other roles in different fish such as an air-breathing organ, a sound producer and a resonator in sound production. In species where it is used as an air-breathing organ (physostomous species), a pneumatic duct connects the caudal end of the esophagus to the swimbladder. In these species the organ has undergone structural and physiological modification to be used as an air-breathing org...
Table of contents
- Cover
- Half Title
- Title Page
- Copyright Page
- Preface
- Table of Contents
- List of Contributors
- 1. Adaptation of Gas Exchange Systems in Fish Living in Different Environments
- 2. Morpho-physiological Divergence Across Aquatic Oxygen Gradients in Fishes
- 3. Swimbladder-Lung Homology in Basal Osteichthyes Revisited
- 4. The Effects of Temperature on Respiratory and Cardiac Function of Teleost Fish
- 5. Oxygen Consumption during Embryonic Development of the Mudskipper (Periophthalmus modestus): Implication for the Aerial Development in Burrows
- 6. Gill Morphological Adjustments to Environment and the Gas Exchange Function
- 7. Behavior and Adaptation of Air-breathing Fishes
- 8. The Osmo-respiratory Compromise in Fish: The Effects of Physiological State and the Environment
- 9. Dissolved Oxygen and Gill Morphometry
- 10. Environmental Influences on the Respiratory Physiology and Gut Chemistry of a Facultatively Air-breathing, Tropical Herbivorous Fish Hypostomus regani (Ihering, 1905)
- 11. Osmoregulatory and Respiratory Adaptations of Lake Magadi Fish (Alcolapia grahami)
- 12. Respiratory Function of the Carp, Cyprinus carpio (L.): Portrait of a Hypoxia-tolerant Species
- 13. Blood Gases of the South American Lungfish, Lepidosiren paradoxa: A Comparison to Other Air-breathing Fish and to Amphibians
- 14. Transition from Water to Land in an Extant Group of Fishes: Air Breathing and the Acquisition Sequence of Adaptations for Amphibious Life in Oxudercine Gobies
- 15. Respiratory Function in the South American Lungfish, Lepidosiren paradoxa
- 16. Respiration in Infectious and Non-infectious Gill Diseases
- 17. Control of the Heart in Fish
- Index
- Color Plate Section